Introduction to Power Electronics in EVs
As we pivot towards a more sustainable energy landscape, electric vehicles (EVs) have emerged as a cornerstone of modern transportation. The role of power electronics in this revolution is paramount. With its ability to efficiently and swiftly control and convert electricity, power electronics provide the essential technological infrastructure that facilitates the widespread adoption and operation of EVs.
In an EV, the core function of power electronics is the effective conversion and control of electrical energy. With the primary power source being the battery pack, a high-voltage direct current (DC) power source, power electronics devices are employed to convert this DC power into the alternating current (AC) the electric motor requires for propulsion. This conversion is achieved using an inverter, a critical power electronics device.
Conversely, during regenerative braking, the motor turns into a generator, producing AC power that needs to be converted back to DC to recharge the battery. Power electronics converters known as rectifiers perform this task.
Power electronics are also indispensable in battery management systems (BMS). These systems oversee the battery pack's health, safety, and optimal usage, ensuring its long lifespan and efficient energy utilization. Sophisticated charging systems regulated by power electronics enable rapid and efficient charging of EV batteries while maintaining their safe operating limits.
Other auxiliary systems in the vehicle, like HVAC systems, lighting, and infotainment, also utilize power electronics for the required voltage and current levels. DC-DC converters are used extensively to step down the high battery voltage to lower levels suitable for these systems.
As power electronics technology continues to advance, so does the performance and efficiency of EVs. Advancements in semiconductor materials, like Silicon Carbide (SiC) and Gallium Nitride (GaN), have led to the development of power electronics devices with higher efficiencies, smaller sizes, and better thermal properties.
Figure 1 shows the scheme of a battery electric vehicle.
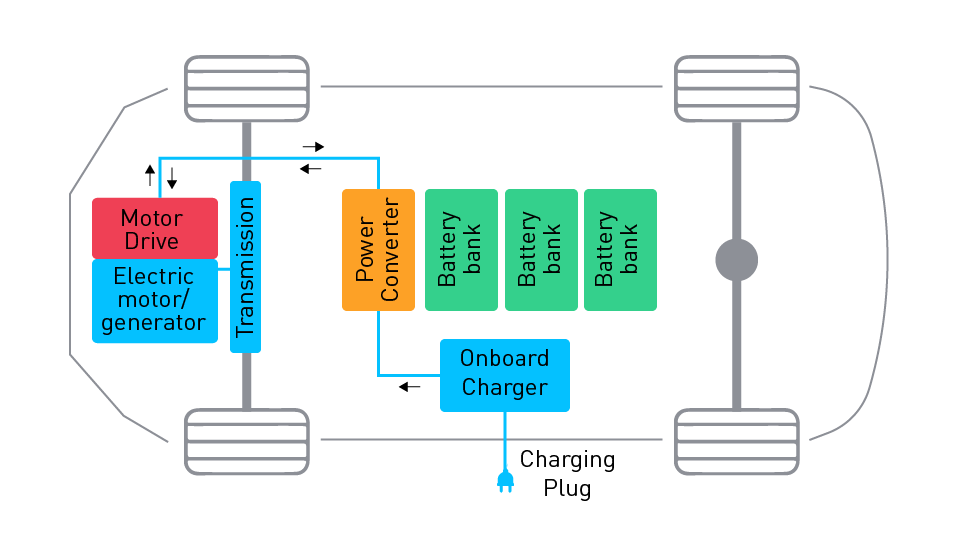
Figure 1: Scheme of a battery electric vehicle
Figure 2 depicts key parts of a battery electric vehicle.
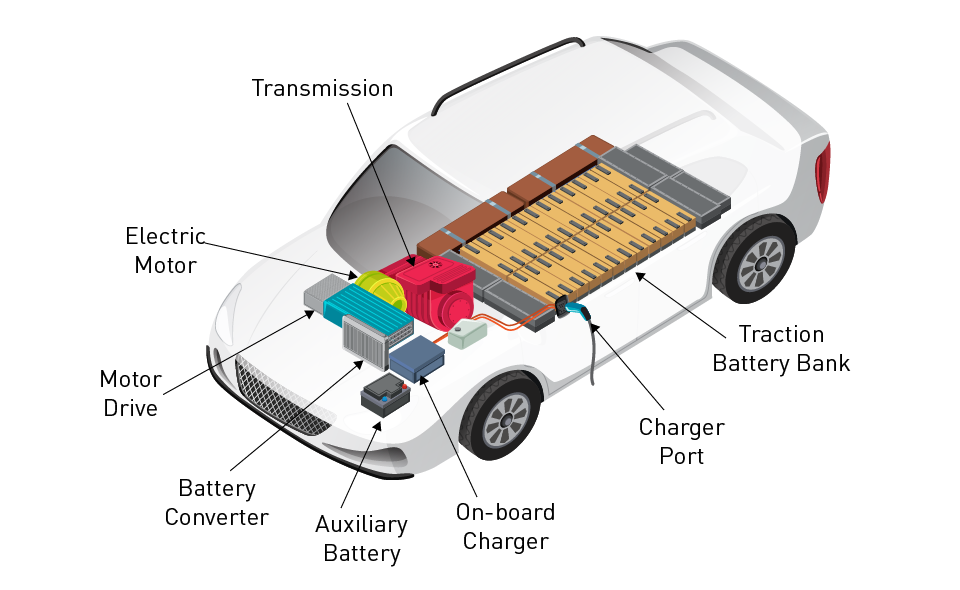
Figure 2: Key parts of a battery electric vehicle
Definitions of the key parts of an EV are as follows:
Charging port or vehicle inlet: It is a connector present on the electric vehicle to allow it to be connected to an external source of electricity for charging.
Power electronic converter: A power electronic converter is made of high power fast-acting semiconductor devices, which act as high-speed switches. Different switching states alter the input voltage and current through the use of capacitive and inductive elements. The result is an output voltage and current, which is at a different level to the input.
On-board charger: It is an AC-to-DC power electronic converter (often referred to as a rectifier) that takes the incoming AC electricity supplied via the charge port and converts it to DC power for charging the traction battery. Using the battery management system, it regulates the battery characteristics such as voltage, current, temperature, and state of charge.
Traction battery pack: It is a high voltage battery used to store energy in the electric car and provide power for use by the electric traction motor.
Battery power converter: It is a DC-to-DC power electronic converter that converts the voltage of the traction battery pack to the higher-voltage of the DC-bus used for power exchange with the traction motor.
Motor drive: It is a DC-to-AC (often referred to as inverter or the variable frequency drive) or at times a DC-to-DC power electronic converter, used to convert power from the high voltage DC bus to AC (or at times DC) power for the operation of motor. The converter is bidirectional for operating in both driving and regenerative braking mode.
Traction electric motor/generator: It is the main propulsion device in an electric car that converts electrical energy from the traction battery to mechanical energy for rotating the wheels. It also generates electricity by extracting energy from the rotating wheels while braking, and transferring that energy back to the traction battery pack.
Transmission: For an electric car, usually a single gear transmission with differential is used to transfer mechanical power from the traction motor to drive the wheels.
Power electronics controller: This unit controls the flow of electrical power in the different power electronic converters in the electric car.
Battery (auxiliary): In an electric drive vehicle, the auxiliary battery provides electricity to start the car before the traction battery is engaged and is also used to power the vehicle accessories.
Role of Power Electronics in Electric Vehicles
In electric vehicle (EV) drive systems, power electronics serve as a critical component in efficiently delivering and controlling electrical energy from the battery to the propulsion unit. In essence, power electronics devices control and convert power, making it available in the appropriate form and quantity when and where it is needed.
EV drive systems typically comprise a battery pack, power electronics converters (including the inverter and DC-DC converter), an electric motor, and control units. The high-voltage DC power from the battery pack needs to be converted into three-phase AC power suitable for the electric motor. This process is facilitated by a key power electronics device, the inverter.
The inverter utilizes pulse width modulation (PWM) techniques to create an AC output waveform from the DC input. Modern inverters also incorporate intelligent control algorithms that allow for variable frequency drive, improving the efficiency and performance of the electric motor .
Conversely, during regenerative braking, the motor functions as a generator. The generated AC power must be converted back to DC power for battery charging. This process of conversion is again facilitated by a power electronics device known as a rectifier.
In addition to the primary power conversion function, power electronics in the EV drive system also enhance efficiency, power factor correction, and reduce harmonics in the electrical system. Advanced power electronics devices built using wide-bandgap materials like Silicon Carbide (SiC) or Gallium Nitride (GaN) are enabling higher efficiencies, compactness, and better thermal characteristics, improving overall vehicle performance.
Power electronics also enable advanced features such as torque vectoring in multi-motor EVs, where individual inverters control each motor, allowing for precise torque control at each wheel. This enhanced level of control can improve vehicle handling, performance, and safety.
Finally, power electronics also play a crucial role in interfacing the vehicle with the grid during charging, including controlling and converting power during both conventional and fast charging scenarios.
Battery Charging Systems and Power Electronics
The efficient and safe charging of the electric vehicle's battery is a central concern in the design and operation of EVs. This process is primarily governed by power electronics systems, which offer control, conversion, and management of electrical energy during the charging process.
Power electronics play a critical role in onboard and off-board EVs' charging systems. In an onboard charger, power electronic converters, including AC-DC converters, are used to convert the AC voltage from the mains to a DC voltage suitable for charging the vehicle's battery. This involves a rectification process followed by a DC-DC conversion stage to provide the required voltage level and control the current flow to the battery. It is essential to ensure that the charging process adheres to the battery's charge profile, which typically involves constant current and constant voltage phases, to preserve the battery's health and longevity.
Off-board charging systems, such as DC fast-charging stations, rely heavily on power electronics. The power electronic converters in these systems convert the AC supply into a high-voltage DC output, which can directly charge the vehicle's battery, bypassing the onboard charger. Advanced control strategies are implemented via power electronics to regulate the charge rate and protect the battery from potential harm.
The advent of bidirectional charging or Vehicle-to-Grid (V2G) technologies has added another layer of complexity and capability to the role of power electronics in EV battery charging. In these systems, power electronics facilitate vehicle charging and the supply of power from the vehicle battery back to the grid or home during peak demand or outages. Bidirectional DC-DC converters are crucial to enabling this two-way power flow, ensuring that power can be efficiently transferred while maintaining the safety and integrity of the grid and vehicle systems.
In wireless charging systems for EVs, power electronics are used to manage the power transfer process between the grid, the primary coil (transmitter), the secondary coil (receiver), and the vehicle's battery. This involves rectification, inversion, and control processes to facilitate efficient power transfer while ensuring safety and standards adherence.
Energy Management and Regenerative Braking
Energy management in Electric Vehicles (EVs) represents a sophisticated orchestration of power flows executed with the assistance of power electronics. This primarily involves distributing power from the battery to various subsystems in the vehicle, such as the electric motor, heating, ventilation, and air conditioning (HVAC) system, and other auxiliary systems. Moreover, energy management includes the recuperation of energy during regenerative braking.
Regenerative braking is a mechanism that enables the conversion of the vehicle's kinetic energy, usually lost as heat during braking, into electrical energy that can be stored in the battery for later use. It is an efficient way to extend the driving range of an EV and reduce the load on the mechanical brakes. Power electronics play a vital role in enabling regenerative braking.
The process begins when the driver applies the brake. The electric motor then acts as a generator, converting the kinetic energy into electrical energy. A power electronic converter, usually an inverter, regulates this process. It controls the energy flow from the motor (acting as a generator) back to the battery.
This operation requires precise control over the inverter to maintain stability, ensuring that the right amount of energy is fed back to the battery while also providing sufficient braking force. The control algorithms running in the background, usually a part of the vehicle's electronic control unit (ECU), have to consider the battery's state of charge and the driver's braking request, among other parameters.
Furthermore, power electronics contribute to the energy management system (EMS) in an EV, which decides the optimal energy distribution from the battery to the different loads in the vehicle. This distribution needs to be dynamic and responsive to changing driving conditions and driver inputs. For instance, more energy should be directed to the drive motor during high-speed driving or rapid acceleration. In contrast, more energy can be allocated to auxiliary systems like HVAC at lower speeds or during idle times.
Power electronic converters, such as DC-DC converters, are used in this context to provide the correct voltage levels and manage power flows to different vehicle subsystems. These converters are controlled by sophisticated algorithms that aim to optimize energy use, extend driving range, and maintain vehicle performance.
Case Studies: Implementations and Innovations in EV Power Electronics
In this section, we explore the real-world implementations and cutting-edge innovations in the field of power electronics for electric vehicles (EVs) through a series of case studies.
Case Study 1: Tesla Model S
As a leader in the electric vehicle industry, Tesla has made significant strides in leveraging power electronics to enhance the performance and efficiency of its vehicles. A key aspect of Tesla's power electronics design is the integrated drive unit, which combines an electric motor, power electronic inverter, and gearbox into a single compact module.
Tesla's inverter employs silicon carbide (SiC) power semiconductors. Unlike traditional silicon devices, SiC devices offer lower losses, higher thermal conductivity, and the ability to operate at high frequencies, translating into smaller, lighter, and more efficient systems. Using SiC power semiconductors in the Model S and Model 3 inverters is a game-changer in terms of increasing efficiency, reducing charging time, and extending range.
Case Study 2: Porsche Taycan
The Porsche Taycan, a high-performance electric vehicle, represents another milestone in power electronics. Porsche uses a two-speed transmission on the rear axle, a rare feature in the EV world, which allows for superior acceleration and a high top speed. The control of this two-speed gearbox is heavily reliant on power electronics.
Furthermore, Porsche's Taycan became the first production EV to use an 800V system instead of the more common 400V system. This innovation doubles the voltage and allows for faster charging and less heat generation. Power electronics, such as DC-DC converters and inverters, had to be carefully designed to handle this high voltage level, ensuring safety and reliability while minimizing losses.
Case Study 3: Wireless EV Charging with WiTricity
WiTricity, a tech company, offers an innovative application of power electronics in the form of wireless charging for electric vehicles. Using resonant inductive coupling, a principle based on Faraday's law of induction, power can be transmitted wirelessly from a charging pad on the ground to a receiver on the vehicle. Power electronics are vital in tuning the resonance between the transmitter and receiver and controlling the power transfer.
This technology offers the convenience of cable-free charging and has the potential to be embedded in public infrastructure for seamless charging as the vehicle is parked or even while in motion.
直接登录
创建新帐号