DC/DC Converters: Common Types, Functionality, Key Parameters And Applications

Get valuable resources straight to your inbox - sent out once per month
We value your privacy
Introduction
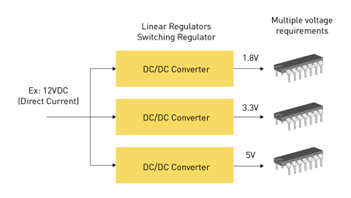
Figure 1: DC/DC converters
DC/DC converters are essential components in many electronic systems, responsible for converting one level of direct current (DC) voltage to another. They are used to regulate and stabilize voltage levels in various electronic systems, ensuring that components receive the appropriate designated voltage for optimal performance and safety as depicted in Figure 1. They play a crucial role in multiple applications such as power supplies, battery chargers, electric vehicles, and renewable energy systems. This article discusses DC/DC converters, their common types, basic operation principles, key parameters, and practical applications.
Types of DC/DC Converters
There are two main variants of DC/DC converters: linear and switching types.
Linear regulators
Linear regulators use linear components e.g. resistors or transistor working in linear region to maintain a constant output voltage by varying the resistance in the conversion path. The regulating circuit continuously varies its resistance, adjusting a voltage divider network to maintain a constant output voltage as illustrated in Figure 2.
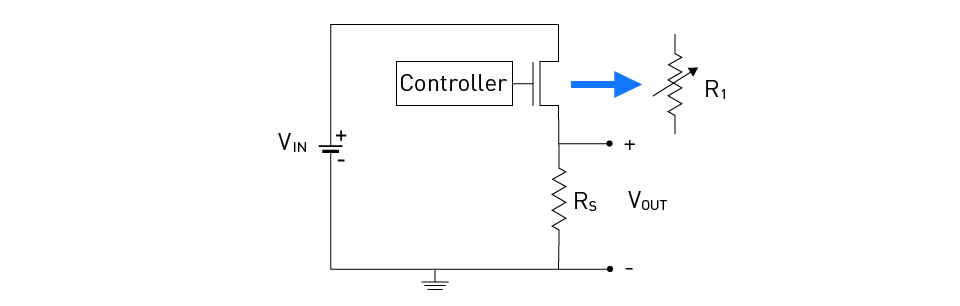
Figure 2: Basic linear regulator circuit
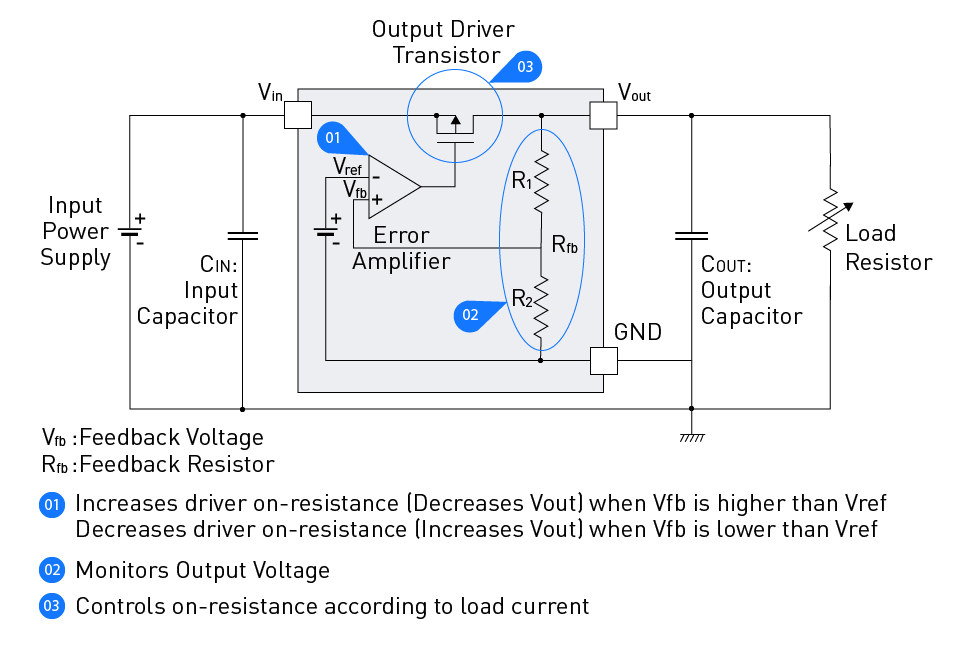
Figure 3: Functionality of linear regulator with feedback.
Commonly feedback to the controller, which can be simple an error amplifier, is provided to maintain the required constant voltage output as depicted in Figure 3. The feedback, in form of stepped down version of output voltage through a resistor divider, is compared to a reference voltage to regulate the output voltage by varying the on-resistance of the output driver transistor. The output voltage (Vo) in terms of reference voltage (Vref) and feedback resistances (R1 and R2) can be written as:
$$ Vo = Vref \times \frac{R1 + R2}{R2} $$In the process, the difference between the input and regulated voltages, known as the dropout voltage, results in wasted energy. Only output lesser than the input voltage is obtainable. Figure 4 depicts how a 3.3V linear regulator consumes 0.17W of the input power of 0.5W during voltage conversion.
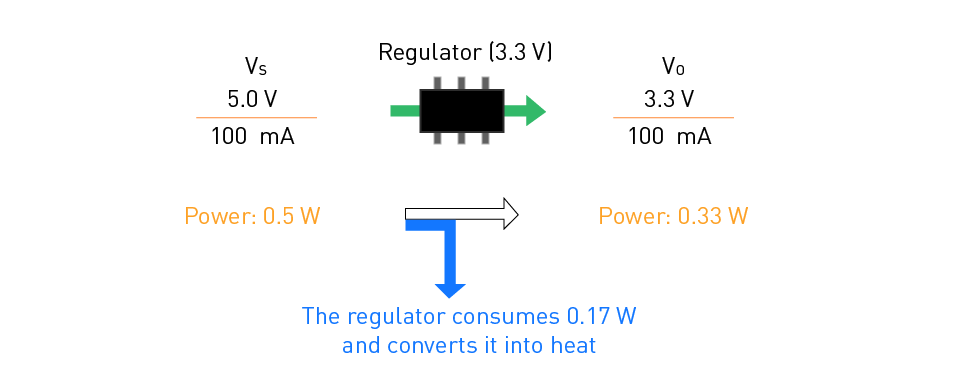
Figure 4: Linear regulation results in wasted energy
Low Dropout (LDO) are variants of linear regulators that can work with lesser drop in voltage during regulation. The pass element in LDOs is commonly implemented with PMOS or NMOS FETs, which, depending on the loading state, can produce a dropout voltage as low as 30 mV to 500 mV.
We now present the key parameters of linear regulators given as follows:
Input Voltage Range: The possible voltage range that can be applied to the input terminal. It must be ensured that the input voltage range guarantees adequate operation or fulfillment of specifications, or if it is a maximum rating value.
Output Voltage Range: Range of output voltages reliably obtainable from the regulator.
Output Accuracy: The extent of error possibly introduced during regulation indicated by +/- %.
Output Current: The current that can be reliably obtained from the output of the regulator.
Dropout Voltage: Voltage difference between input and output necessary to regulate the output. It is also known as the loss voltage or the difference between the input and output voltages. If the difference is still smaller, the regulator stops working correctly.
Transient Response Characteristics: Time to settle the fluctuations of output voltage when rapid load current changes occur e.g. when large-power load wakes up. Transient response characteristics can be verified by plotting empirical data. Both the output capacitance and the IC performance have an impact on transient response characteristics.
Ripple Rejection Ratio: The percentage of ripples contained in the input voltage that is mitigated in the output voltage. It is expressed as dB in most cases. It is sometimes referred to as PSRR (power supply voltage ripple rejection) or input voltage ripple rejection. Note that it depends on the frequency of the ripples.
Quiescent Current: The current required to power the regulator’s internal circuit given the external load current is zero. It comprises the operating currents for the overcurrent and overtemperature detection circuits, the error amplifier, the output voltage divider, and the band-gap reference. The input voltage, temperature, and topology all affect the quiescent current.
Load Regulation: The output voltage change for a given load change. It is typically defined from no load to full load.
Line Regulation: The output voltage change for a given input voltage change. Typically, the full input voltage range is considered to determine the line regulation.
Temperature Rating: This is the maximum temperature that the regulator should not exceed under full load. The regulator may overheat and damage if pushed above this safety limit.
Switching DC/DC Converters
Switching converters use high-speed electronic switches to alternately connect and disconnect the input voltage to an energy storage element, including inductors and capacitors. This process generates a pulsating output voltage, which is then filtered and smoothed to produce a stable output voltage. Switching converters are more efficient and can handle a wider range of input and output voltages but are more complex and generate more noise compared to the linear converters.
Some common types of switching DC/DC converters include:
1. Buck (step-down) Converters
These convert the input voltage from a higher voltage level to a lower output voltage level. The circuit diagram of a basic Buck converter is shown in Figure 5.
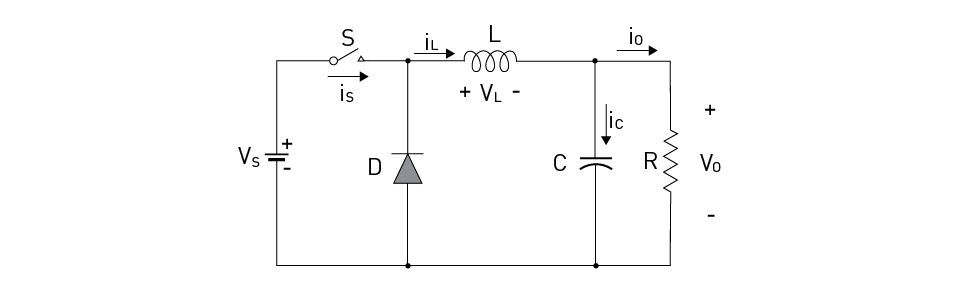
Figure 5: Buck converter circuit
The switch (S) operates at a high frequency. When S is on, the input voltage source (Vs) supplies the current (is) to charge the inductor (L) and capacitor (C) with respective currents (iL and ic). The load (R) is also fed with D being reverse biased and C regulates the output voltage (Vo). On the other hand, when S gets off, the diode becomes forward biased providing iL the path to supply the load current (Io). The inductor and capacitor have to be properly designed to minimize ripple current and voltage in the output.
2. Boost (step-up) Converters
These convert the input voltage from a lower level to a higher output level. The circuit of a basic boost converter is depicted in Figure 6.
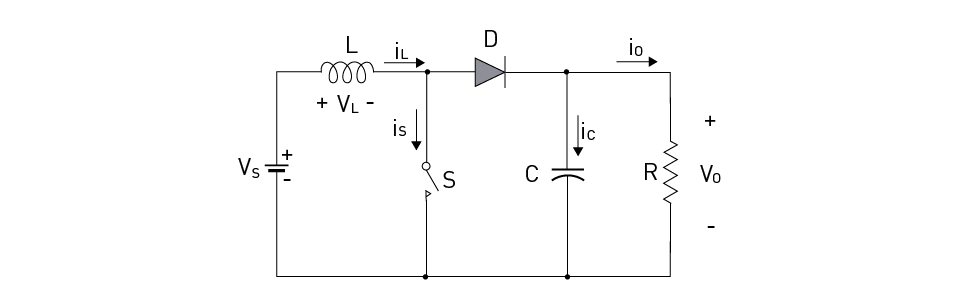
Figure 6: Boost converter circuit
When the switch S is on, the source (Vs) charges the inductor (L) with current (iL) and the switch, carrying current (is), short-circuits the remaining circuit. Whereas when S is off, the input current would now pass through L, the diode (D), the capacitor (C), and the load (R). The currents through C and R are denoted with ic and Io respectively. The energy stored in inductor L is transferred to the load. Up until the next cycle, when S is turned back on, the inductor current decreases.
3. Buck-Boost (step-up/down) Converters
As the name suggests, these combine the step up and step down capabilities of the Buck and Boost converters. This converter is also known as an inverting regulator as the output generated has inverse polarity to the input. The circuit arrangement of a basic Buck-Boost converter is shown in Figure 7.
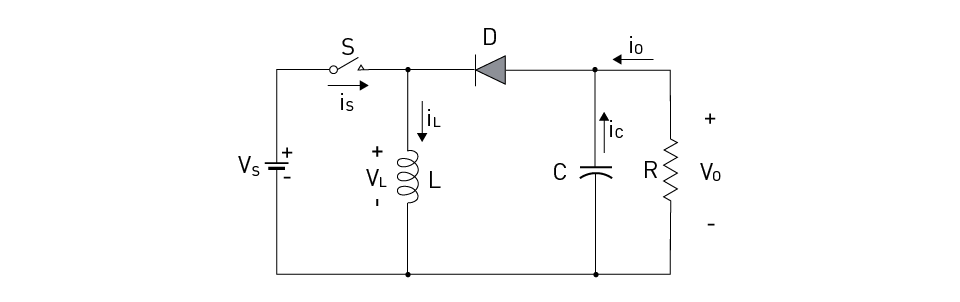
Figure 7: Buck-Boost converter circuit
When the switch (S) is on the input voltage source (Vs) supplies the input current to the inductor (L). On the other hand, when S is off the load (R), the output capacitor (C), and the diode (D) would all receive the current flowing through L. Until S is turned back on in the subsequent cycle, the energy stored in L would be transmitted to the load and the inductor current would decrease. The operating duty cycle controls whether the converter operates in the Buck or Boost mode.
Other Variants
Note that we have presented only the basic switching DC/DC converter topologies. However, there are several other topologies available e.g. as in Cuk, Sepic and Zeta converters. Moreover, the above presented DC/DC converter types do not provide any galvanic isolation between the input and output. There are circuit topologies available that can induce the isolation e.g. transformer variants of these like forward, push-pull, flyback converters etc. In addition, bidirectional converters also exist which allow for a current flow in both directions: from the input source to the load and vice versa.
Switching DC/DC Converters - Associated Concepts and Approaches
Voltage Regulation Methods
It is possible to regulate the output voltage DC/DC converters using different modulation methods, such as pulse-width modulation (PWM) or pulse-frequency modulation (PFM). In PWM, duty cycles are altered while frequency remains constant. The switching losses lower the efficiency because, even under light load situations, the frequency is constant. However, the noise filtering is made easier by the set frequency. On the other hand, in PFM the on- (or off-) time is fixed, whereas the off- (or on-) time is modified. Operations at lower frequencies reduce switching loss. Noise-filtering in PFM is challenging because of the unknown frequency, which causes some noise to appear in an audible band. Figure 8 depicts the difference between PWM and PFM techniques.
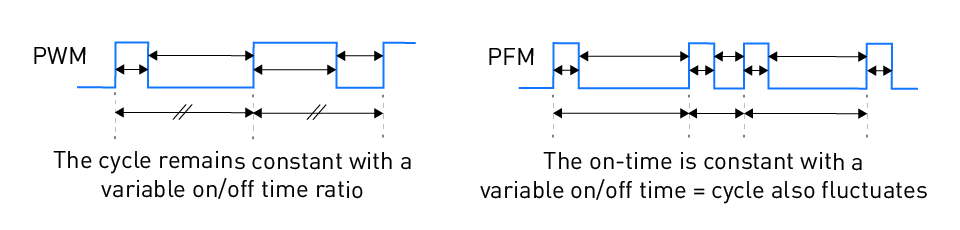
Figure 8: PWM vs PFM
Nonsynchronous/Synchronous converters
Nonsynchronous converters are switching regulators that use a diode as the rectifying element. Figure 9 depicts the nonsynchronous version of the buck converter.
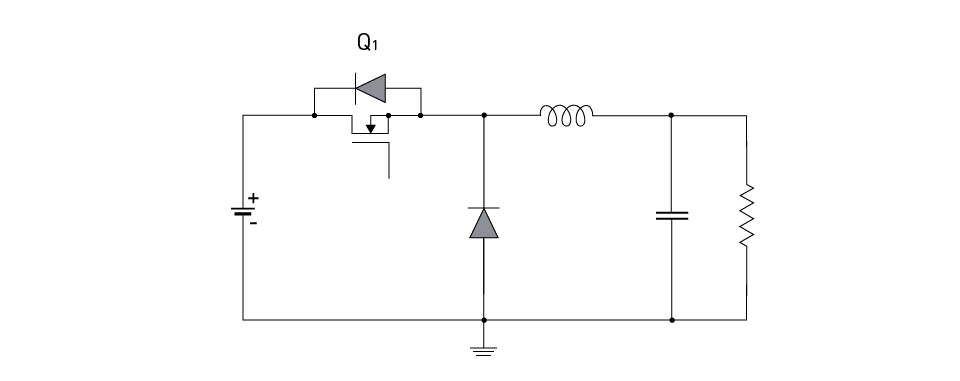
Figure 9: Nonsynchronous buck converter circuits
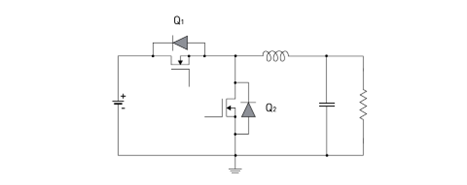
Figure 10: Synchronous buck converter circuit
It should be noted that alternative topologies, such as buck-boost, boost, flyback, etc., can be built as synchronous converters by swapping out the diode as the rectifying element with a transistor. This is advantageous as the forward voltage drop of a diode contributes to a decrease in efficiency. This contribution is especially significant in low output voltage circuitry. However, the enhanced efficiency comes at the expense of more intricate switch driving circuitry. A great precaution must be taken to prevent turning on both switches at once because doing so would short the input voltage source. In order to achieve this, a dead time—also known as a blanking time—must be added to PWM driving signals. The efficiency of synchronous converters is generally higher at optimal operation (~95%) while that of nonsynchronous is lower at optimal operation (~80%).
Control Methods
Control methods in DC/DC converters regulate the output voltage under varying load and input voltage conditions. There are two main control techniques employed to this end namely the voltage mode control, current mode control.
1. Voltage Mode Control
The most fundamental control technique is the voltage mode control, which uses a feedback loop to only return the output voltage as shown in Figure 11. A PWM generator compares triangular waves to the differential voltage, which is obtained by an error amp that compares the output voltage with the reference voltage. As a result, the output voltage is controlled by the PWM signal's pulse width.
This approach has the advantages of being very simple due to the usage of a feedback loop that only uses voltages and having a high noise tolerance. On the other hand, phase compensation circuit complexity and a laborious design approach are the potential downsides.
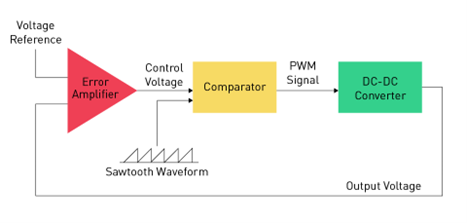
Figure 11: Voltage Mode Control
The current mode is a variation on voltage mode control where the triangle waveforms used in voltage mode control are replaced by the inductor current or the on-resistance of a high-side MOSFET in the circuit as shown in Figure 12. The control is rather sophisticated since the current mode has two different types of feedback loops: voltage loop and current loop.
The advantages of the current mode include a significantly simplified phase compensation circuit design and a quicker load transient response than the voltage mode. Due to the great sensitivity of current sensing, there is a disadvantage of low noise tolerance. However, in more recent designs, the current detection component is integrated into the IC to address the issue.
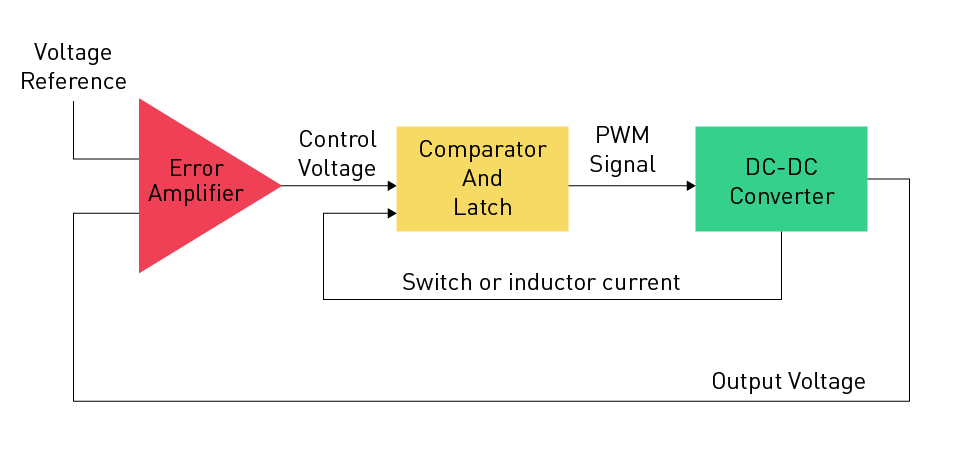
Figure 12: Current Mode Control
Hysteretic (or bang-bang) control is another form of dc-dc converter control strategy that is the simplest to implement in hardware. Without using an error amp, the method uses a comparator to directly check the output voltage. The comparator directly activates or deactivates the switch when it determines that the output voltage has reached or fallen below a predetermined threshold level. Due to the direct control the comparator exerts and no need for phase compensation, this approach provides the benefit of very quick transient responses. However, the hysteretic control results in a variable frequency of operation for semiconductor switch.
2. Soft-Switching Techniques
The basic converters described above are devised with hard-switching approaches. An advanced technique known as the soft switching method is also possible in DC-DC converters. It refers to the elimination of the turn-on or turn-off switching losses in one or more power switches. This is in contrast to hard switching, where both turn-on and turnoff of the power switches are done at high current and high voltage levels.
We now present the important parameters of switching DC/DC converters given as below:
- Efficiency: The ratio of output power to input power, indicating how effectively the converter can transfer in the input power.
- Load and line regulation: The ability to maintain a stable output voltage under varying load and input voltage conditions, respectively.
- Transient response: The converter's ability to respond to sudden changes in load or input voltage.
- Voltage rating: It dictates the limits on how far up or how far down the DC-DC converters can transform a voltage.
- Current rating: The maximum amount of current that is safely obtainable DC-DC converter provide to a load. Even if the DC-DC converter is able to supply greater current, it may overheat and malfunction.
- Temperature rating: This is the maximum temperature that the DC-DC converter should run in under full load. The DC-DC converter may overheat and sustain damage if pushed above this safety limit, or it may shut down as a safeguard.
- Ripple voltage: It is important to consider how much ripple appears in the input/output voltages of the regulator. It must be ensured that the regulator’s voltage ripple rating meets the requirements.
MPS provides a powerful DC/DC designer suite to design a power converter depending on the requirements.
The user can analyze design performance including steady state, load transient, loop analysis, efficiency etc. The online version of the designer can be accessed here.
The comparison of salient features of linear and switching DC/DC converters is summarized in Table 1.
Table 1: Comparison of linear and switching DC/DC converters
Linear Regulator | Switching Regulator | ||||
Buck Boost Buck/Boost Inverting |
Possible Impossible Impossible Impossible |
Possible Possible Possible Possible |
|||
Efficiency |
VO / VIN Mostly low |
Approx. 95% Usually high |
|||
Output Power |
Generally several watts Depending on thermal design |
Large power possible | |||
Noise | Low | Switching noise exists | |||
Design | Simple | Complicated | |||
BOM | Low count | High count | |||
Cost | Low | Relatively high |
Applications
Applications of DC/DC converters are ubiquitous ranging from automotive, domestic electronics, mobile phones, smart watches, robotics etc. These are used to provide the optimal voltage levels in the circuitry of the devices. Both the linear and switching converters can be leveraged depending on the requirements and determining which regulator better fulfil the requirements. Sometimes these converters can be used in tandem. For example, to minimize the ripples in the output of switching DC/DC converters, linear regulators can be used for further regulation.
Conclusion
DC/DC converters play a critical role in a wide range of electronic systems, providing stable voltage levels for optimal performance and safety of the underlying circuitry. We covered the two fundamental types of converters, linear and switching converters, as well as their principles of operation, important design parameters, comparison, and practical applications of DC/DC converters, which can serve as a useful introduction to the subject for the readers.
_______________________
Did you find this interesting? Get valuable resources straight to your inbox - sent out once per month!
直接登录
创建新帐号