Passive vs. Active
Within the broad category of sensor technologies, sensors are principally categorized according to their mode of operation and energy consumption. The distinction between passive and active sensors is one basic classification that is frequently essential to comprehending sensor dynamics.
Definition and Distinction
Passive Sensors: For these sensors to function, there is no need for an external power source. Rather, they depend on outside energy or modifications to the physical surroundings to produce a quantifiable reaction; in other words, the sensor transforms the energy of the input stimulus into the signal that is produced. Examples include photodiodes, which convert light into voltage, thermocouples, which convert temperature variations into voltage, and piezoelectric sensors, which generate a voltage in response to mechanical stress.
Active Sensors: Active sensors need an external power source, in contrast to passive sensors. Usually, they alter the surroundings, then track how the changes are received. A typical example is radar, which uses electromagnetic waves emitted by a transmitter module and waves reflected by a receiver module to measure an object's distance and speed.
Energy Sources for Each Type
Passive Sensors: Passive sensors are intrinsically powered by the variable they are intended to detect. For example, the energy source for a photodiode (used to measure light intensity) is incoming light, whereas the thermocouple gets its energy from the temperature gradient it is monitoring.
Active Sensors: These sensors require external power sources to function, which might be wired electrical supplies in fixed installations or batteries in portable devices. Certain situations, such as the radar example, involve the sensor emitting energy (in this case, electromagnetic waves) that interact with the surroundings, and the sensor then measures the interaction that results.
Advantages and Disadvantages is Various Applications
Passive Sensor Advantages
- They are perfect for isolated or difficult-to-reach places because they don't require external power.
- Often more robust due of their simpler designs.
- Since they don't generate energy that could ignite volatile substances, they can be intrinsically safe in hazardous conditions.
Passive Sensor Disadvantages
- A lower signal-to-noise ratio may result from external, unpredictable influences affecting their output.
- May not be appropriate for dynamic or real-time measurements in settings that change quickly.
- Cannot determine a target's distance, speed, or direction.
Active Sensor Advantages
- Can be more accurate because the signal that is released is controlled.
- Capable of functioning in situations with minimal or no light.
- Appropriate for applications that demand quick responses or real-time data.
- Considering their energy source, they frequently have a wider range of observable properties.
Active Sensor Disadvantages
- Reliance on outside energy sources may restrict their use in isolated locations.
- Occasionally, the energy released might cause errors by interfering with other systems or being tampered with.
- Are easier to detect, making them more susceptible to countermeasures.
- In general, more complex, which could lead to higher costs and lower durability.
Ultimately, the selection between passive and active sensors is contingent upon the particular application, its goals, the level of precision required, and the resources at hand. Additionally, integrating or merging various sensor kinds might improve a system's capabilities. For engineers and designers to choose the right sensors for their projects, they must have a thorough understanding of these classes.
Absolute vs. Relative
The two types of sensors are absolute and relative, depending on which reference is used.
Definition
Absolute Sensors: Measurement circumstances are irrelevant for detecting stimuli as compared to an absolute physical scale. A temperature sensitive resistor, or thermistor, is an illustration of an absolute sensor. There exists a clear correlation between the electrical resistance of the sensor and the Kelvin temperature scale.
Relative Sensors: Generate a reference-related signal. Thermocouples, which measure temperature by producing an electric voltage based on the gradient in temperature across their wires, are an example of a relative sensor.
Criteria to Choose Between Absolute or Relative Sensors
The needs of the application ultimately determine which sensor is best. Since absolute sensors yield exact readings without the need for reference points, they are perfect for situations where precise and clear measurements are crucial. They could have smaller measurement ranges, nevertheless, and are typically more expensive. For applications where pressure monitoring is required that is unaffected by temperature variations, an absolute sensor would be appropriate.
When changes or differences in values relative to a reference point are sufficient, relative sensors, on the other hand, are more affordable, have wider measurement ranges, and are frequently chosen. Applications requiring pressure measurement relative to air pressure, for instance, can benefit from the use of a relative sensor.
Analog vs. Digital
Sensors are essential in the current era of electronics and information technology. The distinction between analog and digital sensors is notable among the several classifications because it has a fundamental effect on signal processing, transmission, and integration into more complicated systems.
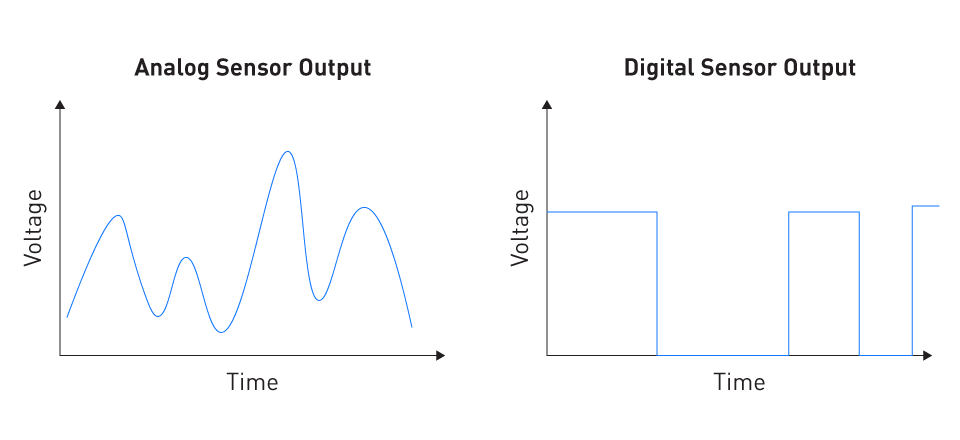
Figure 1: Analog vs. Digital Sensors
Analog Sensors: Continuous Signals and Their Interpretations
Analog Sensors: An output signal from an analog sensor is typically a continuous signal that represents the observed attribute exactly. A constant voltage, current, or resistance that varies proportionately with the change in the measured parameter could be the signal. For example, when employed as a temperature sensor, a standard thermistor changes resistance in response to temperature, producing an analog signal.
Interpretation: Components or analog circuitry capable of capturing the continuous subtleties of the signal are necessary for reading and understanding analog signals. Analog waveforms are frequently visualized with oscilloscopes, for instance. Signal degradation over long distances and susceptibility to noise, interference, and distortion are two of the main issues with analog sensors.
Digital Sensors: Discrete Signals, Sampling, and Quantization
Digital Sensors: Digital sensors, in contrast to their analog counterparts, provide discrete or digital output signals. This usually entails a conversion procedure that turns the analog signal, which is produced by the sensor's natural interaction with the environment, into a sequence of binary values (0s and 1s).
Sampling and Quantization: Converting continuous analog impulses to discrete digital signals involves two key steps:
- Sampling: Sampling is the process of periodically capturing "snapshots" of the analog signal at a specific frequency, which is referred to as the sample rate.
- Quantization: Assigning a digital value to every sampled analog data point is the process of quantization. Particularly if the quantization levels are not precise enough, this may result in some information loss.
Transitioning from Analog to Digital: Implications for Signal Processing and IoT
Signal Processing: Once digital signals are collected, these can be processed with the help of digital electronics, which takes use of the energy efficiency, compactness, and robustness of contemporary integrated circuits. Due to this, complex algorithms, filtering strategies, and other DSP techniques that could be difficult or ineffective in the analog domain can be implemented.
Internet of Things (IoT): Digital sensors are replacing analog ones, and this has significant effects for the Internet of Things. Digital communication modules, microcontrollers, and other digital devices can readily interface with digital sensors. Through this smooth integration, sensors can now send data over networks, participate in big data analytics, and work together to create intelligent, networked ecosystems that enable real-time data processing and decision-making.
Although analog sensors offer distinct benefits and remain essential in some scenarios, the global trend is gradually shifting towards digital sensing. This change is expected to improve accuracy, increase integration capacity, and pave the way for new applications in areas like Industry 4.0, smart cities, and the Internet of Things.
Quantitative vs. Qualitative
Within the field of sensor technology, sensors are differentiated not only by the sort of signal they produce or how much energy they consume, but also by the type of data they offer. This classification can be broadly divided into quantitative and qualitative categories, each of which has a distinct function and provides particular insights into the systems it monitors.
Quantitative Sensors
Definition: Sensors that measure, count, or compare numerical values on a scale are known as quantitative sensors. The exact numbers that these sensors provide are frequently within a predetermined range. Examples include light sensors that provide lux values, pressure sensors that detect precise pressure values, and temperature sensors that provide precise temperature readings.
Applications: In scientific research, control systems, and any other situation where precise measurements are essential, quantitative sensors are widely used. For instance, precise measurement of a machine's pressure or temperature in an industrial context may be essential to its safe operation.
Qualitative Sensors
Definition: Non-numerical information is provided by qualitative sensors, however. They offer descriptive or category data as opposed to exact measurements. These sensors may identify patterns, categorize data into large groups, or show the presence or absence of a given condition. An example of this would be a smoke detector, which simply signals the presence of smoke rather than its exact quantity.
Applications: When merely identifying or detecting a state is sufficient, qualitative sensors are especially helpful. Systems that rely more on qualitative data than accurate measurements include security systems, environmental monitoring systems, and setups for pattern recognition.
Balancing Quantitative Sensors and Qualitative Sensors in Multi-Sensor Systems and Advanced Applications
Both quantitative and qualitative sensors must be integrated in many contemporary applications, particularly in complex configurations like multi-sensor systems. Although qualitative sensors offer more comprehensive insights or can be used to trigger specific actions when certain criteria are met, quantitative sensors provide the granularity and precision needed for precise control and analysis.
In a sophisticated agricultural system, for example, quantitative sensors track temperature, nutrient concentrations, and soil moisture levels, while qualitative sensors look for particular pests or illnesses. All together, these sensors offer an all-encompassing perspective of the farm, facilitating both targeted agricultural techniques and comprehensive interventions as required.
Furthermore, the combination of quantitative and qualitative data can be extremely useful in the era of machine learning (ML) and artificial intelligence (AI). In addition to being trained to make predictions or choices based on precise measurements from quantitative data, algorithms can also be trained to identify patterns from qualitative data.
Although they have diverse uses, quantitative and qualitative sensors frequently work well together. When selecting the appropriate combination of sensors for a particular application, it is crucial to comprehend the type of data needed and the insights that are being pursued.
直接登录
创建新帐号