Thyristor Basics
The thyristor, commonly referred to as a silicon-controlled rectifier (SCR) is a semiconductor device that possesses unique characteristics that render it highly suitable for a diverse range of power electronics applications.
Thyristor Structure
A thyristor is a semiconductor device that has three terminals and four alternating layers of p-type and n-type materials arranged in a p-n-p-n configuration. This creates three PN junctions (J1, J2, and J3) within the device. The three terminals are the anode, cathode, and gate, each connected to one of the device's layers. The anode should be connected to the outermost p-type layer, the cathode to the outermost n-type layer, and the gate to the inner p-type layer. The p-n junctions of a thyristor are illustrated in Figure 5, while Figure 6 depicts the commonly used symbol for a thyristor.

Figure 5. Thyristor p-n junctions

Figure 6. Thyristor symbol
Thyristor Operation
The functioning of a thyristor can be understood by analyzing the behavior of the three PN junctions under varying voltage conditions. When a positive voltage is applied to the anode with respect to the cathode, junctions J1 and J3 become forward-biased, while junction J2 becomes reverse-biased. In this state, the thyristor does not conduct current and remains in the off or blocking state. The thyristor can be activated by increasing the applied voltage between the anode and cathode to a high value, causing the reverse-biased J2 junction to break down in a process known as avalanche breakdown. This method however is harmful to the transistor, to turn on the thyristor safely, a positive voltage is applied between its gate and cathode, which causes the breakdown of junction J2 at a lower applied voltage due to the behavior of holes and electrons across junctions. The gate pulse injects minority carriers into the reverse-biased junction J2, neutralizing the depletion region and enabling current flow through the device. The thyristor remains in the ON state once the anode current exceeds the latching current, as long as the anode current does not fall below the holding current. To turn off the thyristor, the anode current must be reduced below the holding current, which returns the device to the blocking state. An important aspect of thyristor operation is that increasing the gate current reduces the forward-blocking voltage, allowing the thyristor to be activated with a lower anode-to-cathode voltage by increasing the gate current. It is crucial to remove the gate signal once the thyristor has turned on to prevent any unnecessary power losses. Similarly, there should not be any gate signal during reverse-biased conditions, as this can lead to device failure due to increased leakage current. It should be noted that special gate circuitry is necessary to trigger the thyristors and ensure isolation between the power and control circuitry for protection. Usually, isolation circuits such as pulse transformers or optocouplers are employed to achieve this.
Thyristor Properties
Thyristors possess distinct characteristics that set them apart from other semiconductor devices, such as diodes and transistors. These features encompass the ability to block high voltage, carry high current, and exhibit low conduction losses, rendering them appropriate for high-power applications. Also, thyristors are known for their superior reliability, durability, and cost-effectiveness in comparison to other power electronic devices.
Thyristor Characteristics
The v-I characteristics of a thyristor are illustrated in Figure 7.
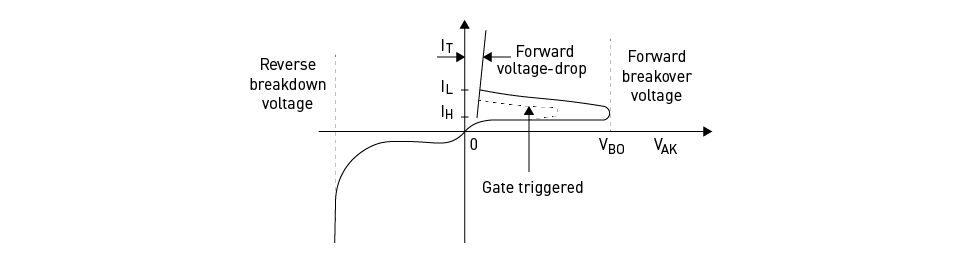
Figure 7. Thyristor V-I characteristics
Forward Breaking Voltage (VBO)
The forward breakdown voltage (VBO) refers to the voltage level at which the J2 junction begins to conduct or the avalanche breakdown takes place, without the need for a gate signal to be applied between the anode and cathode.
Latching Current (IL)
The determination of the latching current is of utmost importance in the design of circuits using thyristors. This parameter guarantees the maintenance of the thyristor in its ON state under the intended operating conditions, immediately after the removal of the gate signal. Failure to consider the appropriate latching current may lead to unreliable turn-on, resulting in suboptimal performance or malfunction. Conversely, a thyristor with a low latching current may be susceptible to unintended operation due to noise or transient current spikes.
Holding Current (IH)
The holding current is the minimum anode current below which the thyristor will turn OFF and revert to its blocking state. Once the thyristor is in the ON state and the anode current falls below the holding current, the thyristor will turn off and not conduct current until turned on again. The holding current is a key parameter in designing thyristor-based circuits, as it helps ensure stable operation and prevents the thyristor from unintentionally turning off during normal operation.
Turn-on Time (ton)
The turn-on time (ton) is the time required for the thyristor to conduct electricity after the application of a gate pulse. Specifically, the ton is defined as the duration between the point at which the steady-state gate current reaches 10% (0.1IG) and the point at which the thyristor on-state current reaches 90% (0.9IT). Ton can be calculated as the sum of the delay time (td) and the rise time (tr), where td is the time between 10% of the gate current (0.1IG) and 10% of the thyristor on-state current (0.1IT). The rise time (tr) is the duration required for the anode current to increase from 10% of the on-state current (0.1IT) to 90% of the on-state current (0.9IT).
Thyristor Types
Various types of thyristors exist based on the unique characteristics that differentiate them from the fundamental phase-controlled thyristor, also known as the SCR.
Bidirectional Phase-Controlled
Thyristor (BCT) combines the benefits of two antiparallel thyristors into a single package, resulting in a compact design, simplified cooling systems, and heightened reliability. BCTs are equipped with two gates that regulate the forward and reverse current and are commonly employed in applications such as volt-ampere reactive (VAR) compensators, static switches, and motor drives.
Bidirectional Triode Thyristors
Bidirectional triode thyristors are also referred to as TRIACs, they are composed of two antiparallel thyristors. Notably, the gate connection is shared in this bidirectional device. These components are frequently employed in AC phase control circuits.
Gate Turn-off Thyristors (GTOs)
The GTO, or Gate Turn-Off Thyristor, can be activated with a positive gate signal like an SCR. However, the unique aspect of GTOs is that they can be deactivated with a negative gate signal. The activation process for GTOs is less reliable than for SCRs, and a small positive gate current must be maintained even after activation for optimal performance. GTOs are utilized in high-power inverters and variable-speed drives.
Other Thyristor types
Additional variations of thyristors exist, such as light-activated silicon-controlled rectifiers (LASCRs). Also, certain thyristors integrate field-effect transistors (FETs) and metal-oxide-semiconductor field-effect transistors (MOSFETs), such as FET-controlled thyristors (FET-CTHs) and MOS turn-off thyristors (MTOs).
Thyristor Applications
Thyristors are used in several electrical applications, they include:
AC power control: regulating power in heating, lighting, and motor control systems.
Thyristors are a commonly utilized component in AC power control applications due to their ability to efficiently manage large amounts of power.
In heating systems, thyristors can be employed to regulate temperature by controlling the power delivered to resistive heating elements. By manipulating the firing angle of the thyristor, the power delivered to the heating element can be modulated, thereby maintaining the desired temperature.
In lighting systems, they are used to regulate the brightness of lamps, such as incandescent or halogen bulbs, by controlling the power delivered to the lamps. This method of control is referred to as phase control dimming.
In motor control systems, thyristors are utilized to regulate the speed and torque of AC motors, such as induction or synchronous motors. The thyristor-based motor control system can precisely manage the motor's speed and torque by adjusting the voltage and frequency applied to the motor.
Phase-controlled rectifiers: Converting AC to DC in high-power applications
Phase-controlled rectifiers are electronic circuits that utilize thyristors to convert high-power AC power into DC power. Compared to traditional diode-based rectifiers, phase-controlled rectifiers offer superior control over output voltage, enhanced efficiency, and reduced harmonic distortion. The thyristors in a phase-controlled rectifier are triggered at a specific phase angle during each half-cycle of the AC input waveform, allowing for the adjustment of the DC output voltage to a desired level by controlling the firing angle. This feature makes phase-controlled rectifiers particularly well-suited for applications that require variable DC voltage, such as electric vehicle chargers, battery charging systems, and high-voltage DC transmission systems.
Inverters: Converting DC to AC for motor drives and renewable energy systems
Thyristor-based inverters are commonly employed in various applications, including motor drives and renewable energy systems, to convert DC power into AC power.
In motor drives, the inverter generates an adjustable frequency and voltage AC output to regulate the motor's speed and torque. Due to their ability to handle significant amounts of power, thyristor-based inverters are frequently utilized in high-power applications, such as industrial drives and locomotive traction systems.
In renewable energy systems, such as solar and wind power installations, the generated power is typically DC, which must be converted to AC using an inverter to integrate it into the grid or power AC loads. Thyristor-based inverters can be utilized in these systems to produce grid-compatible AC power with lower harmonic distortion and higher efficiency.
直接登录
创建新帐号