Introduction to Adaptive and Robust Control
In power electronic systems, control strategies play a crucial role in achieving desired performance, efficiency, and reliability. Traditional control methods, such as Proportional-Integral-Derivative (PID) controllers, may not always provide satisfactory results, especially when the system experiences parameter variations, nonlinearity, or external disturbances. To address these challenges, adaptive and robust control techniques have been developed, offering improved performance and increased resilience under various operating conditions.
Adaptive control techniques are designed to adjust their parameters or structure in real-time based on the system's behavior, compensating for changes in the system or its environment. This adaptability allows the control system to maintain optimal performance even in the presence of uncertainties or disturbances. Model Reference Adaptive Control (MRAC) and self-tuning control are examples of adaptive control strategies.
On the other hand, robust control techniques focus on maintaining system stability and performance in the presence of uncertainties, disturbances, or parameter variations, without the need for adaptation. The main objective of robust control is to design a controller that guarantees a specified level of performance for all possible variations within a given range. H-infinity control and sliding mode control are well-known examples of robust control methods.
Both adaptive and robust control techniques offer several advantages in power electronic systems, such as improved transient response, enhanced steady-state performance, and increased resilience against uncertainties and disturbances. These control strategies can be applied to various power electronic applications, including voltage and current regulation, motor drives, renewable energy systems, and grid-connected inverters.
In the following sections, we will discuss the principles and techniques of adaptive and robust control in greater detail, focusing on their applications in power electronic systems.
Adaptive Control Techniques
Adaptive control approaches dynamically adjust the controller parameters to accommodate changes in the system parameters and external disturbances. The fundamental premise behind these techniques is the ability to modify the control strategy based on the feedback from the system to minimize the error between the desired output and the actual output. The two commonly used adaptive control strategies in power electronic systems are Model Reference Adaptive Control (MRAC) and Self-tuning Control.
Model Reference Adaptive Control (MRAC)
Model Reference Adaptive Control (MRAC) is a method where the controller is designed to force the system to follow a desired model. The model represents the ideal response of the system. An adaptive law is used to adjust the controller parameters based on the difference between the system output and the reference model output, commonly known as the tracking error.
The goal of MRAC is to make the actual system follow the reference model as closely as possible, regardless of any changes in the system parameters or external disturbances. This is accomplished by adjusting the controller parameters in real-time to minimize the tracking error. One advantage of MRAC is its ability to handle system uncertainties or nonlinearity, making it a suitable control strategy for power electronic systems with varying parameters or operating conditions.
The block diagram of model reference adaptive control of uninterruptible power supply is depicted in Figure 1. In this figure, vinv, v0, v0,ref, if and i0 denote output voltage of the inverter, the capacitor voltage, the reference input voltage, the inverter/inductor current and load current, respectively. In addition, v0,m and if,m are the output voltage and current of the reference system, while if,ref denotes the reference current input. The adaption parameters are denoted as k1, k2, k3, and k4, while the voltage and current tracking errors are denoted as ev0 and eif.
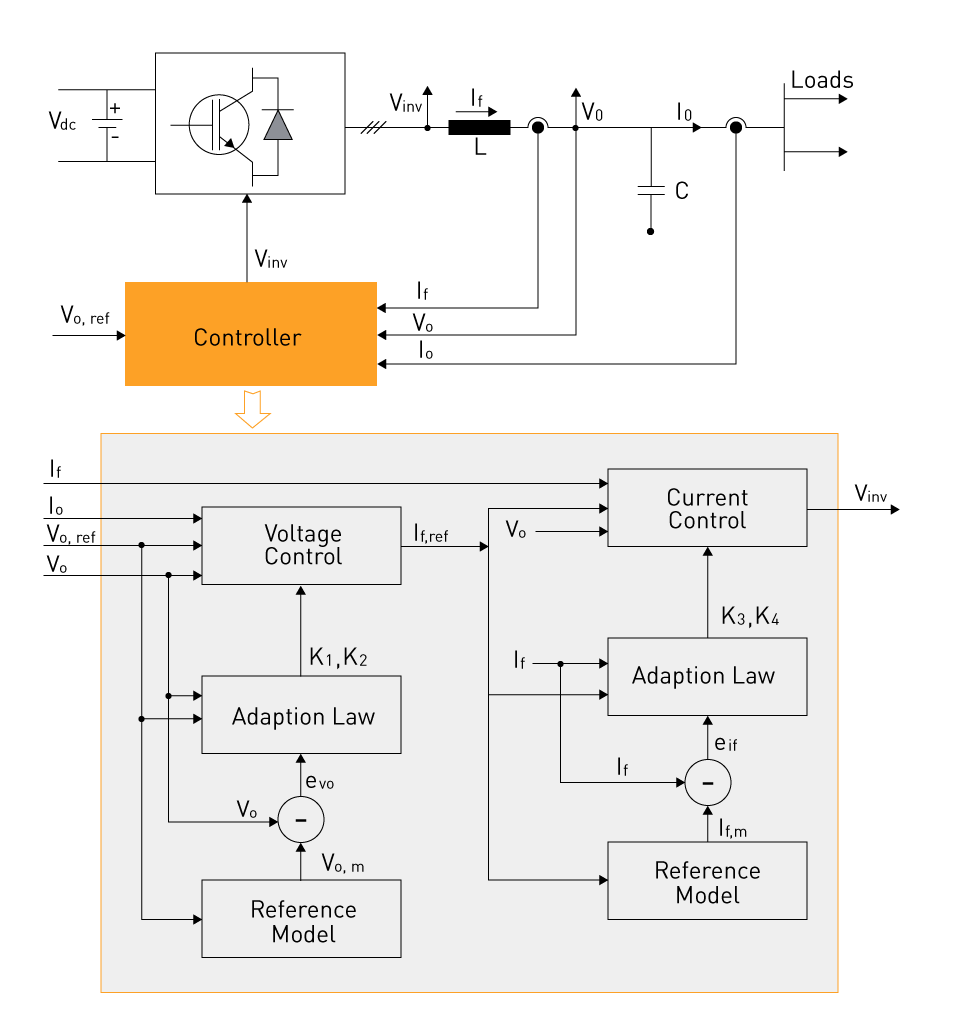
Figure 1: Block diagram of model reference adaptive control of uninterruptible power supply
Self-Tuning Control
Self-tuning control, another form of adaptive control, automatically adjusts the controller parameters based on the observed performance of the control system. This method uses an online identification process to estimate the system parameters, which are then used to update the controller parameters based on a pre-defined optimization criterion.
In power electronic systems, self-tuning control can effectively deal with system nonlinearities and uncertainties. It is especially useful when system parameters are not precisely known or vary over time. The primary advantage of self-tuning control lies in its ability to maintain optimal control performance despite changes in system dynamics.
MRAC and self-tuning control techniques can significantly improve the performance and reliability of power electronic systems, especially under varying operating conditions or in the presence of uncertainties. The choice between these techniques depends on the system's specific requirements, such as the level of system uncertainties, the desired performance characteristics, and the computational resources available for implementing the control algorithm.
Robust Control Techniques
Robust control techniques are designed to maintain the stability and performance of a control system in the presence of uncertainties and disturbances. They provide an effective way to handle system parameter variations and unmodeled dynamics, common issues in power electronic systems. Among the various robust control strategies, H-infinity control and sliding mode control are widely used due to their superior performance and robustness properties.
H-infinity Control
H-infinity control is a robust control method used to design controllers to achieve robust performance and stability. The main objective of the H-infinity control method is to minimize the maximum gain of the system's transfer function from the disturbance input to the output error, from infinity to negative infinity, thus giving it the name H-infinity. This results in a system that is less sensitive to uncertainties and provides improved performance over a wide frequency range.
The H-infinity control is highly effective in managing uncertain or varying system dynamics, making it well-suited for power electronic systems. However, it requires the system to be represented in the form of linear, time-invariant differential equations, and the design and implementation of H-infinity controllers can be complex due to the requirement of solving a set of nonlinear matrix inequalities.
Sliding Mode Control
Sliding mode control is a nonlinear control method that can provide robustness against system uncertainties. In sliding mode control, a sliding surface is defined in the state space of the system, and the control law is designed to drive the system states onto this surface. Once on the surface, the system is constrained to move along it, resulting in a reduced-order system insensitive to matched uncertainties and disturbances.
One of the key advantages of sliding mode control is its robustness to parameter variations and external disturbances. This makes it highly suitable for power electronic systems, where the system parameters can change due to varying operating conditions or component aging. However, implementing sliding mode control can be challenging due to the so-called "chattering" phenomenon, which is high-frequency oscillation in the control signal caused by the discontinuous control law. Various methods, such as the boundary layer approach or high-order sliding mode, have been proposed to alleviate this issue.
Applications in Power Electronic Systems
Adaptive and robust control techniques have found extensive applications in power electronic systems due to their ability to handle uncertainties and offer stable and efficient system performance. This section will discuss some of the notable applications of these techniques in power electronics.
DC-DC Converters: Adaptive and robust control strategies, such as Model Reference Adaptive Control (MRAC) and H-infinity control, are often employed in DC-DC converters to regulate the output voltage under a wide range of operating conditions and load variations. These techniques ensure stable operation and optimal performance, even in parameter uncertainties and external disturbances.
AC-DC Rectifiers: AC-DC rectifiers can benefit from robust control techniques like sliding mode control to manage input current harmonics and improve power factors. Such techniques help provide a robust performance against parameter variations and load disturbances, ensuring the reliable operation of the rectifiers.
DC-AC Inverters: In DC-AC inverters, adaptive control techniques like self-tuning control are used to regulate the output voltage and frequency. These techniques can adapt to changes in system parameters and operating conditions, providing efficient and reliable performance.
Electric Drives: Electric drive systems, including AC and DC drives, are a prominent application area for adaptive and robust control techniques. These techniques help precisely control motor speed, torque, and position, even in the presence of varying loads and parameter uncertainties.
Renewable Energy Systems: Adaptive and robust control techniques are used to maximize power extraction under varying environmental conditions in renewable energy systems, such as solar photovoltaic (PV) systems and wind energy systems. These techniques help optimize the performance of maximum power point tracking (MPPT) algorithms and power converters used in these systems.
直接登录
创建新帐号