Matrix Converters
Matrix converters are a type of sophisticated AC/AC converter that converts an input AC voltage to an output AC voltage with configurable amplitude, frequency, and phase. Unlike standard AC/AC converters, which include intermediary DC linkages and energy storage components, matrix converters use a grid of bidirectional, controlled power semiconductor switches to perform direct conversion. This section will cover the fundamentals, operation, and practical implications of matrix converters.
Fundamentals Of Matrix Converters
A matrix converter is made up of a matrix of bi-directional power switches that link the input and output phases. A three-phase to three-phase matrix converter includes nine bi-directional switches, each of which is commonly made up of two back-to-back linked semiconductor devices, such as IGBTs or MOSFETs, with anti-parallel diodes (Figure 7).
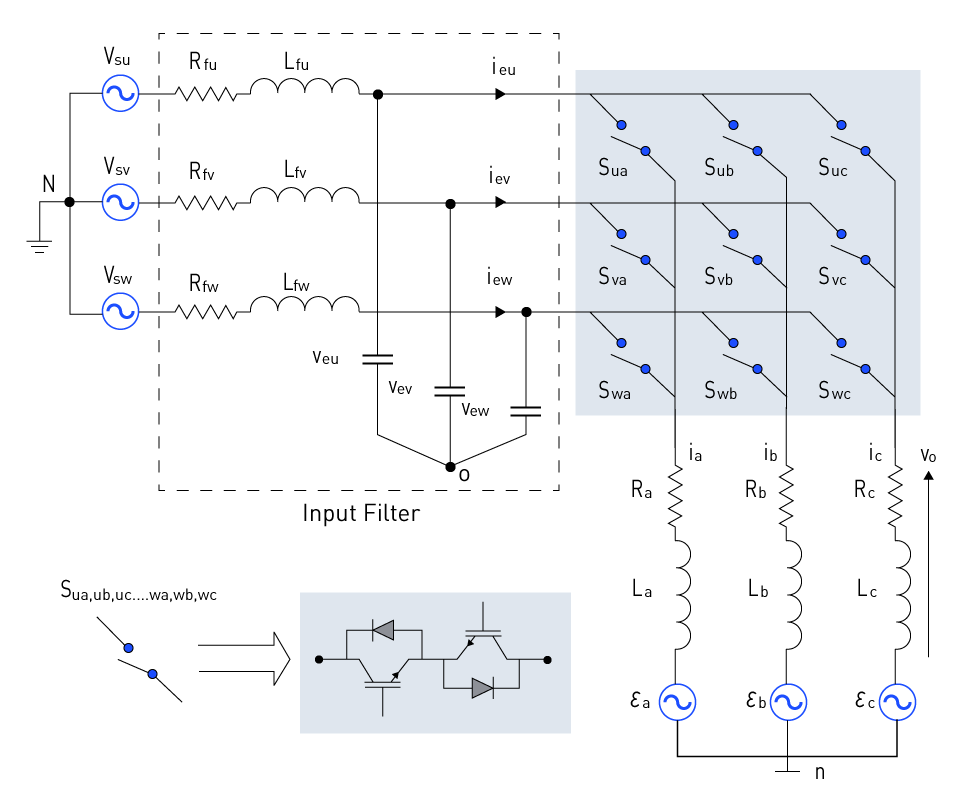
Figure 7: Matrix converter topology
Operation Of Matrix Converters
The matrix converter transfers power from the input phases to the output phases by selectively shutting and opening the bi-directional switches. The converter may generate an output voltage waveform with the required amplitude, frequency, and phase shift by adjusting the duty cycle and timing of the switches. The switching operation also affects the input current waveform, keeping it sinusoidal and in phase with the input voltage, resulting in a high power factor.
Advantages And Challenges:
Matrix converters provide various benefits over standard AC/AC converter topologies, including
- Due to the lack of energy storage parts and direct power conversion, the efficiency is high.
- Compact design and lower component count because no bulky DC link capacitors or inductors are required.
- Excellent input power factor control and fast dynamic response.
However, matrix converters have certain drawbacks:
- The control algorithm is complicated because it demands perfect coordination of the switching states to achieve the required output voltage and input current waveforms.
- Shoot-through faults can occur when two switches in the same row or column close simultaneously, resulting in a short circuit across the input or output phases.
- A three-phase matrix converter has a limited output voltage range, generally up to 86.6% of the input voltage.
Applications Of Matrix Converters
Matrix converters have found use in a variety of fields, including motor drives, renewable energy systems, and power quality enhancement. These offer accurate speed and torque control of AC motors in motor drive applications, with the added benefits of a compact design and higher efficiency. Matrix converters can be used in renewable energy systems to connect distributed energy resources, such as wind turbines or solar panels, to the power grid, allowing for flexible power conversion and management.
Soft-Switching Techniques
Soft-switching methods are used in AC/AC converters to decrease switching losses and electromagnetic interference (EMI) associated with hard-switching operation. Hard-switching is the process of turning on or off a power semiconductor switch while both current and voltage are non-zero, resulting in high energy loss and increased stress on the device. Soft-switching approaches try to reduce or eliminate these effects by maintaining zero voltage or current during switching transitions. This section discusses the principles of soft-switching, the primary soft-switching approaches, and their consequences for converter performance and design.
Fundamentals Of Soft-Switching
There are two types of soft-switching techniques: zero-voltage switching (ZVS) and zero-current switching (ZCS).
Zero-Voltage Switching (ZVS): When a power switch is switched on or off, ZVS procedures ensure that the voltage across it is zero. ZVS dramatically minimizes switching losses and device stress by reducing voltage and current overlap during the switching transition. It is especially useful in high-voltage, high-frequency applications, where switching losses can account for a large amount of overall losses.
Zero-Current Switching (ZCS): When a power switch is switched on or off, ZCS procedures ensure that the current flowing through it is zero. This decreases switching losses and EMI production caused by rapid changes in current. ZCS is especially useful in high-current applications, where switching losses might be significant.
Main Soft-Switching Techniques
To accomplish ZVS or ZCS functioning in AC/AC converters, a number of soft-switching approaches have been devised. Among the most important techniques are:
Resonant converters: These converters use resonant tank circuits, which are made up of inductors and capacitors, to generate resonant transitions that cause the voltage or current to be zero during the switching operation. Resonant converters may provide greater efficiency and low EMI, but sophisticated control techniques and bigger passive components may be required.
Auxiliary circuits: To provide soft-switching circumstances, auxiliary circuits also known as snubber circuits or active clamp circuits, are added to the primary converter architecture. Additional switches, inductors, and capacitors are generally included in these auxiliary circuits to shape the voltage and current waveforms, enabling ZVS or ZCS operation.
Implications On Converter Performance And Design
Implementing soft-switching methods may considerably improve the efficiency, EMI, and device stress of AC/AC converters. However, these advantages are not without drawbacks:
Increased complexity: Soft-switching approaches sometimes need more complicated control schemes and extra components, increasing the total complexity and expense of the converter.
Component selection and rating: These approaches may place unique demands on converter components, such as fast-switching devices, low-loss capacitors, or high-quality inductors.
Stability and transient response: The integration of resonant components or auxiliary circuits might impact the stability and transient response of the converter, necessitating careful study and design.
Resonant Converters
Resonant converters are a type of power electronic converter that uses resonant circuits to modify the voltage and current waveforms. These are responsible for soft-switching operation and lowering the switching losses associated with standard hard-switching topologies.
The converters offer high efficiency, decreased electromagnetic interference (EMI), and increased performance in a variety of applications by combining resonant components such as inductors and capacitors. This section discusses the fundamental concepts of resonant converters, their major topologies, the design considerations and problems connected with these converters.
Fundamentals Of Resonant Converters
The usage of resonant tank circuits, which consist of inductors and capacitors coupled in series or parallel, is the basic principle in resonant converters. At particular resonant frequencies, these resonant devices generate oscillating voltage and current waveforms, ensuring zero-voltage switching (ZVS) or zero-current switching (ZCS) operations. Soft-switching allows resonant converters to reduce switching losses, improve efficiency, and reduce stress on power semiconductor devices.
Main Resonant Converter Topologies
In AC/AC conversion applications, a variety of resonant converter topologies can be used. The following are some of the most prevalent topologies:
Series Resonant Converters (SRC): The SRCs link the resonant tank circuit to the load in series, and the converter runs at or near the series resonant frequency. These may operate in ZVS mode and provide strong output voltage control.
Parallel Resonant Converters (PRC): The resonant tank circuit is linked in parallel with the load in PRCs, and the converter runs at or close to the parallel resonant frequency. PRCs may operate in ZCS mode and have minimal output current ripple.
Series-Parallel Resonant Converters (SPRC): The SPRCs combine series and parallel resonant circuits, utilizing both SRCs and PRCs. These converters can provide improved efficiency and output voltage regulation, but they may require more sophisticated control techniques.
Design Considerations And Challenges
There are various specific concerns and obstacles in the design of resonant converters, including:
Component selection: The selection of resonant inductors and capacitors is crucial in order to achieve the optimum converter performance. These components must have minimal losses and be able to withstand the voltage and current pressures of the converter.
Resonant frequency control: The resonant frequency control is critical for the soft-switching operation and output voltage regulation of the converter. Complex control algorithms and real-time monitoring of the resonance frequency may be required.
Stability and transient response: Complex dynamic behavior in resonant converters can impair the converter's stability and transient responsiveness. To guarantee steady operation under varying load situations, designers must assess the converter's small-signal and large-signal performance.
Sensorless Control Strategies
Sensorless control techniques are a new field of study in power electronics. These strategies arrived with the goal of improving the performance, reliability, and cost-effectiveness of AC/AC converters by cutting-off the requirement for specific sensors. Sensors are used in traditional control systems to monitor and regulate converter characteristics like voltage, current, phase angle, and frequency. These sensors, however, can add complexity, expense, and possible failure points to the system.
Sensorless control strategies estimate these values by using the converter's intrinsic electrical qualities and dynamic reactivity, thus, eliminating the requirement for separate sensing devices. This section covers the fundamentals of sensorless control techniques, their advantages and disadvantages, and their use in AC/AC converters.
Fundamentals Of Sensorless Control
Sensorless control techniques predict the necessary system parameters through the converter's input and output signals or by utilizing the intrinsic electrical characteristics of the converter components. Sensorless control mechanisms that are often employed include:
Model-based methods: To determine the needed parameters, these strategies depend on mathematical models of the converter system. State observers, such as Kalman filters or sliding mode observers, are frequently used in model-based approaches to estimate system states and parameters in real time.
Signal injection techniques: In these procedures, a known signal is injected into the converter system and the resultant response is observed to determine the appropriate parameters. This can be accomplished by modulating the control signals of the converter or by directly inserting a high-frequency signal into the circuit.
Parameter estimation techniques: These methods estimate the needed parameters by examining the input and output signals of the converter, frequently using advanced signal processing techniques such as Fourier analysis, wavelet transformations, or adaptive filtering algorithms.
Advantages And Challenges
Sensorless control systems provide various advantages over typical sensor-based control approaches. These advantages include:
Reduced cost: Sensorless control techniques can lower the total cost of the converter system by removing the necessity for specialized sensors.
Improved reliability: With the help of sensorless control strategies, it is possible to mitigate potential failure points and improve the overall credibility and robustness of the converter.
Simplified design: Sensorless control approaches do not require a number of components and linkages, thereby, assisting in simplifying converter design.
Nevertheless, there are certain drawbacks to employing sensorless control strategies:
Complexity: Sensorless control methods sometimes entail complicated algorithms that need large computing resources, thereby increasing the cost and complexity of the converter's control system.
Robustness and accuracy: These techniques may be more susceptible to parameter fluctuations, noise, and disturbances, thereby impacting the control system's accuracy and resilience.
Transient response and stability: Sensorless control schemes' dynamic performance could be different from that of standard sensor-based systems, thereby compromising the converter's transient responsiveness and stability.
Application In AC/AC Converters
Sensorless control techniques have been used effectively to a variety of AC/AC converters, including phase-controlled converters, cycloconverters, and matrix converters. These strategies may be employed to estimate characteristics like phase angle, frequency, or load impedance, allowing for better converter performance and control.
直接登录
创建新帐号