Introduction to Power Distribution
One of the fundamental components of automotive systems that guarantees the effective movement of electrical energy inside the car is power distribution. Sturdy and effective power distribution networks are more important than ever because of the rise of electric and hybrid cars and their increasingly sophisticated technical features. The purpose of power distribution in automobile power management is explained in this introduction, along with the difficulties that contemporary power distribution systems face.
Role of Power Distribution in Automotive Power Management
One way to think of power distribution is as the electrical components of a vehicle's circulatory system. It carries out several vital tasks:
Transmission of Energy: Engine controls, lighting, entertainment, and safety systems are just a few of the subsystems that get power via power distribution networks, which transport electricity from batteries, alternators, or fuel cells.
Voltage Regulation: Making sure the right voltage, taking into account different operating circumstances and load demands, reaches the right components.
Efficiency Management: This is the process of maximizing energy flow to reduce losses and raise total vehicle efficiency.
Integration with BMS: To regulate the flow of electricity to and from the battery in electric and hybrid cars, the electricity Distribution System (PDS) and Battery Management System (BMS) must collaborate closely.
Scalability: The capacity to quickly add or change electrical subsystems, allowing for flexibility in response to emerging features or technologies.
Challenges in Power Distribution
Power distribution in contemporary cars has several difficulties due to their complexity:
Complex Loads: A single power distribution component is frequently built to meet the demands of many systems with varying voltage and current needs, which may fluctuate dynamically depending on the operating environment.
Weight and Space Constraints: The necessity for a lot of wiring and components might make the car heavier and take up a lot of room.
Reliability: It's critical to maintain a steady power supply even in the face of environmental variables including dampness, temperature swings, and vibration.
Electromagnetic Interference (EMI): Controlling electromagnetic interference (EMI) is necessary to avoid interference between various electrical systems. Particularly in parts of the power distribution system like on-board chargers and DC-DC converters, which when switching hundreds of amperes produce a lot of noise.
Compliance with Standards: Adhering to a number of safety and regulatory requirements, especially those related to high-voltage systems in electric cars, including IEC 60071.
Cost Considerations: Creating a reliable and effective PDS at a reasonable cost is a never-ending engineering problem.
In contemporary automotive systems, power distribution is essential for the dependable and effective transfer of electrical energy to the many parts that make up a car. The deployment of electric and hybrid powertrains, the increasing complexity of vehicle electronics, and the need for greater dependability and economy have all added layers of complexity to the planning and operation of power distribution networks.
To overcome these obstacles, engineers must have a thorough grasp of materials science, electrical engineering concepts, and automobile design specifications. The relevance of this essential component of automotive engineering will be highlighted by the expected increase in innovation and sophistication in the field of vehicle power distribution as technology develops.
Architecture of Power Distribution Systems
The design of power distribution systems plays a key role in automotive power management since it dictates how electrical energy is distributed to different parts and subsystems inside a car. To meet the many and frequently intricate needs of contemporary automobile systems, the architecture must be strong, versatile, and adaptive. The primary categories of power distribution architectures and their component parts are examined in this section.
Centralized, Distributed, and Hybrid Power Distribution
Centralized Power Distribution
In centralized power distribution, the delivery of electrical power to different subsystems is managed by a single control point or unit. This method lowers the number of possible failure spots and streamlines the design.
- Pros: Less complicated, easier to build, and less wiring.
- Cons: Less ability to adjust to changing requirements; a single failure may impact the entire system.
- Example: The usage of a central fuse box is a well-known illustration of centralized power distribution in earlier vehicle systems. Here, just one hub provides electricity to every electrical component in the car. Usually, you'd wire an auxiliary device straight to this central fuse box if you wanted to add one (such as an extra pair of headlights or an updated audio).
Distributed Power Distribution
Multiple distribution points or units are dispersed throughout the vehicle in distributed power distribution, which decentralizes control. This can boost dependability and flexibility.
- Pros: Enhanced fault isolation, increased scalability, and enhanced flexibility to accommodate varying load demands.
- Cons: More difficult to maintain and design, and possible weight increases from adding more components.
- Example: To improve the safety of their advanced driver assistance systems (ADAS), modern cars frequently use a dispersed power distribution strategy. To power the modules in each zone, for instance, a zone design distributes the power distribution boxes such that each zone has a separate power distribution unit. Better control over the power distribution system is made possible by the semiconductor switches used in the smart power distribution units rather than relays and fuses.
Hybrid Power Distribution
Aspects of distributed and centralized systems are combined in hybrid power distribution. This combination frequently aims to minimize the shortcomings of each system while maximizing its benefits.
- Pros: Personalized adaptability, harmony between dependability and intricacy.
- Cons: May be difficult to optimize.
- Example: A hybrid power distribution approach is used by many modern electric cars (EVs). The major powertrain and core vehicle systems may be centrally supplied by the main high-voltage battery; however, distributed smart semiconductor junction boxes may be included to manage features like window controls, seat heating, and cabin lighting. This gives flexibility for supplementary operations and potential future updates, as well as effective usage of the primary battery.
Fuses, Relays, Contactors and Solid-State Components in Power Distribution
Fuses
To prevent harm to other components, fuses serve as safety devices that interrupt the circuit if the current exceeds a certain limit.
- Types: These include resettable, EV, cartridge, and blade fuses.
- Applications: Protection against overcurrent in different circuits.
Within fuse boxes are blade fuses, cartridge fuses, and EV fuses that guard connections and the vehicle harness from overheating in the event of a short circuit. The cable and connections that the fuse is intended to protect must have current ratings that match the fuse hold current rating chosen.
On the circuit boards of ECUs, resettable fuses, or PTCs as they are more often called, guard against overcurrents at the inputs and outputs of the circuit.
Relays
Relays are electrically driven switches that isolate high-voltage and low-voltage domains or enable control of a high-power circuit with a low-power signal.
- Types: These include Reed relays and electromechanical relays.
- Applications: These include power windows, sunroofs, fuel pump activation, headlight control, and engine control modules.
An electromagnetic coil used in electromechanical relays may be used to switch the armature contactor by moving it from one position to another.
In a glass tube, reed relays include magnetic contact materials that will make contact with a magnetic field. When it comes to position sensing in cars, particularly for comfort features, they are an affordable option.
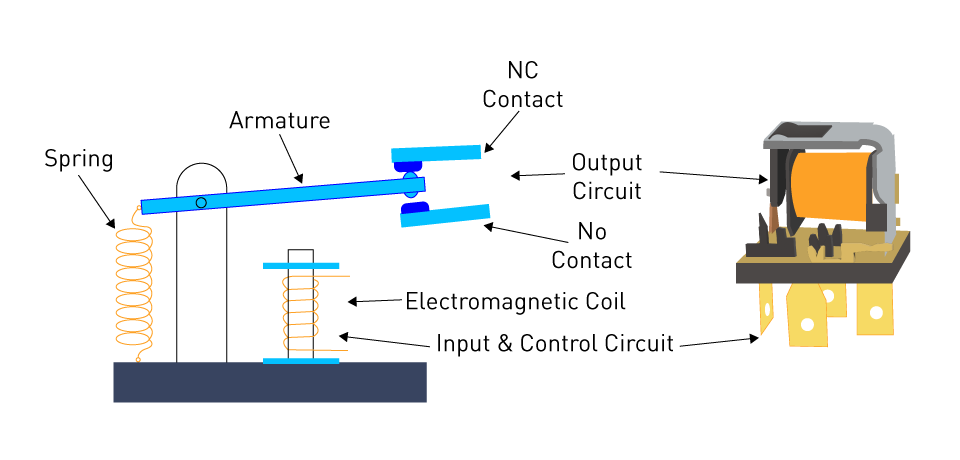
Figure 1: Electromechanical Relay
Reed relays have magnetic contact materials in a glass tube, that when subjected to magnetic field, will make contact. They are a cheap solution when it comes to position sensing in vehicles, mostly in the comfort functions.
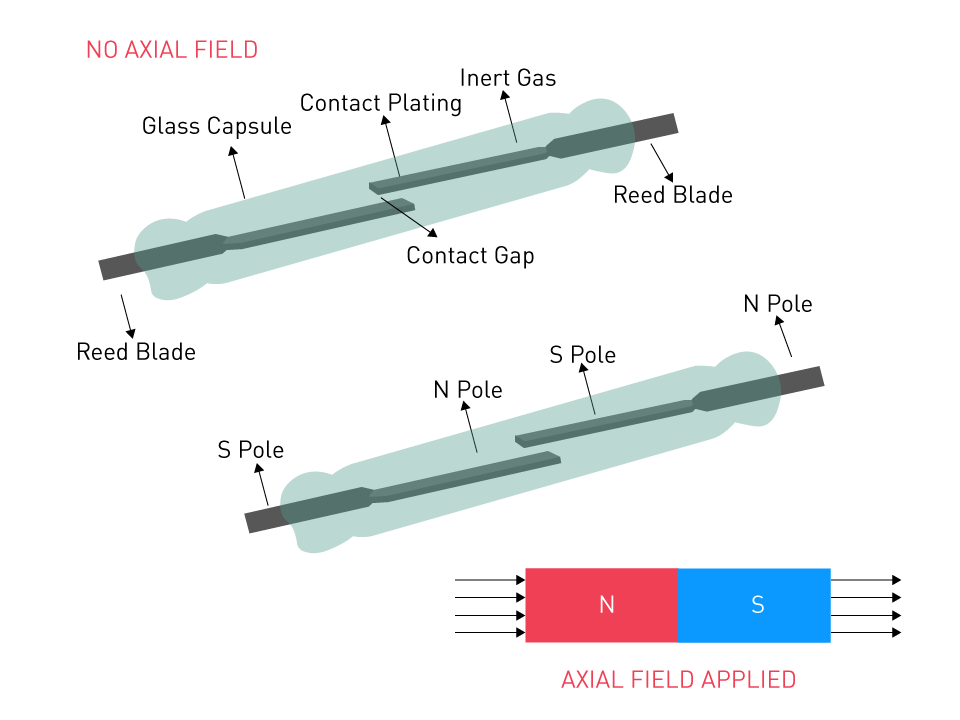
Figure 2: Reed Relay
Contractors
In electric cars, contactors, special relays made to regulate high current loads are frequently utilized to control the connection between the battery, the e-machine, and the charging grid. Contactors frequently have the ability to switch and interrupt current at thousands of amps.
- Types: DC contactors, AC contactors.
- Applications: High-power battery management, motor control.
Semiconductor Switches
Compared to relays, which have switching periods in the tens of milliseconds, semiconductor switches, such as MOSFETs and IGBTs, provide for accurate and efficient management of electrical power, especially in applications requiring high-speed switching. Semiconductors can switch in nanoseconds.
- Types: MOSFETs, IGBTs, Triacs.
- Applications: Includes DC-DC converters, e-PDUs, onboard chargers, and electric motor drives.
Power distribution architecture in automotive systems is a complicated field that necessitates careful consideration of design elements including complexity, scalability, efficiency, and dependability. The way a vehicle's electrical system operates and adjusts to different operating situations is determined by the choice of centralized, distributed, and hybrid designs as well as the careful selection and usage of parts like fuses, relays, contactors, and solid-state devices.
Automotive engineers may design power distribution systems that satisfy the demanding specifications of modern cars by grasping these basic ideas. This will ensure smooth functioning across a variety of features and improve the driving experience overall.
Power Distribution Modules (PDMs)
Power Distribution Modules (PDMs) are an essential part of today's automobiles' electrical systems. Within the automobile infrastructure, these devices oversee the distribution and management of electrical power to different subsystems. The fundamental functions and characteristics of PDMs, as well as how they integrate into automotive systems, will be explained in this section.
Role and Features of PDMs
Role of PDMs
PDMs are in charge of obtaining power from the source (such as the battery) and allocating it to the several loads found in the car, including the infotainment systems, lights, sensors, and actuators. They assist in:
- Distribute Power: Channel electricity to several loads with different voltage and current needs in an efficient manner.E
- Protect the System: Put safety measures in place to safeguard the system, such as short-circuit, overcurrent, and voltage protection.
- Facilitate Diagnostics: Information on system performance and fault circumstances should be provided to facilitate diagnostics.
Features of PDMs
Several important characteristics improve the functioning and design of PDMs, including:
- Scalability: The capacity to adjust to various system setups and sizes.
- Flexibility: Allowing different kinds of loads and voltage levels.
- Fault Tolerance: Isolating defects to stop a system-wide failure is known as fault tolerance.E
- Energy Efficiency: This is the process of minimizing losses and maximizing electricity usage.
- Communication Capability: Connecting to other car systems to provide synchronized monitoring and control.
- Compact Design: Making the least amount of space possible, especially in the cramped quarters of cars.
Integration of PDMs in Automotive Systems
Types of PDMs
We only found low-voltage PDMs in automobiles with traditional combustion engines. The vehicle level fuses, relays, and rectifier diodes that ensure proper power distribution to the low-voltage system components are all found in these fuse boxes. The driver can reach the fuse boxes for servicing.
The power distribution modules in EV and HE cars with high-voltage systems are not designed for driver access. For instance, the module in Tesla models is rather difficult to reach because it is concealed beneath the e-machine. EV fuses and smart electronics that monitor the flow of high-voltage power to customers are found in high-voltage distribution modules.
Placement and Connection: PDM integration in an automobile system requires consideration of several aspects, including electromagnetic compatibility, accessibility, temperature control, and weight distribution. To reduce losses and interference, connections between the power supply and loads need to be properly arranged.
Interface with Control Systems: PDMs frequently communicate with other control units, such as Battery Management Systems (BMS) and Engine Control Units (ECUs). To facilitate coordinated operation, this interaction needs reliable communication protocols and real-time data transmission.
Automotive electrical systems rely heavily on Power Distribution Modules (PDMs), which strike a balance between complexity, efficiency, safety, and adaptability. Together with a wide range of characteristics, their complex function in power distribution and management enables them to meet the complex needs of contemporary automobiles.
Automotive engineers may contribute to the overall performance, dependability, and safety of the vehicle by designing and implementing PDMs that are resilient and inventive by understanding the ideas presented here.
Electrical Wiring and Connectors
Electrical wiring and connection design and execution are critical components of contemporary car power distribution systems. They link different parts of the car and act as channels for the transfer of energy. The complexities of wire harness design, connection types, and dangerous voltage concerns in automobile systems will all be covered in this section.
Wiring Harness Design Considerations
Bundles of wires and connectors called wiring harnesses make it easier for electricity and electrical information to be sent throughout the car. Important factors in their design are as follows:
Routing: Organizing the wiring's physical path to reduce interference with other parts and systems.
Protection: Using insulation and shielding to guard against external elements including heat, moisture, and abrasion.
Weight and Size: Creating the harness with space and weight restrictions in mind. Accessibility: Making certain that vital joints and connectors are reachable for upkeep and inspection.
Standardization and Compatibility: Complying with industry guidelines to guarantee that different components work together.
Redundancy: Adding fallback routes for critical operations to improve dependability.
Types of Connectors and Their Applications
Specialized connections called connectors enable the joining and severing of electrical circuits. There are several types of connections used in automobile systems:
Terminal Connectors: They are frequently used in power distribution because they offer strong connections for high current carrying between wires and terminals or bus-bars.
Board-to-Board Connectors: These connectors, which allow connections between several PCBs (printed circuit boards), are frequently seen in control units.
Weatherproof Connectors: Used in outdoor applications, such as for components under the hood, these connectors are specifically made to endure exposure to the elements. The protection level of these connections is often certified at IP68 or IP69, depending on where they are packaged inside the car. The components located beneath the engine may be subjected to immersion in water and hot jet wash cleaning throughout their lifetime; thus, these connectors need to be rated for both IP68 and IP69 protection.
Customer Connectors: The client may access several ports within the cockpit, including the USB port and the cigar lighter.
For optimal performance and dependability, the right connector must be chosen, taking into account aspects like environmental resistance, voltage level, current rating, and simplicity of connection/disconnection.
Hazardous Voltage Considerations
The popularity of hybrid and electric cars has made managing high-voltage systems crucial. Several factors need to be taken into account:
Cable Insulation Selection: Specialized insulating materials that can tolerate high voltages without failing are needed for high-voltage cables in order to safeguard the system and service staff. Insulation standards like ISO 6469 - Electrically powered road vehicles — Safety characteristics are used in tandem with these requirements.
Cable Coloring Requirements: High-voltage lines are frequently distinguished from other cables by their orange color. The correct handling and connection are ensured by this visible indication.
Connector Pin Spacing Requirements: The distance between connection pins in high-voltage applications needs to be big enough to avoid arcing. The minimum distances that must be kept are determined by standards and laws.
Accessibility of Hazardous Voltage Parts in the Vehicle: For safety, it is essential to make sure that high-voltage components are contained or protected and may only be accessed with specific equipment or keys. Usually, warning signs and clear labeling are used. The HVIL (High Voltage Interlock Loop) circuit must be implemented by all high-voltage components. The wiring in this circuit runs between the modules. The design ensures that the HVIL loop is disconnected if a high-voltage cable is unplugged or a high-voltage component is opened. The battery management system detects this disconnection and eliminates the safety risk by cutting off the high-voltage battery contactors.
Electrical wiring and connection design and selection in automobile systems are intricate processes that need careful planning and knowledge of several technical, environmental, and safety factors. Engineers may build reliable and effective power distribution paths that satisfy the many requirements of contemporary cars by abiding by industry standards, safety laws, and good design principles. Hazardous voltage issues require special attention, as evidenced by the changing automobile technological environment and the growing popularity of electric and hybrid vehicles.
Fail-Safe Mechanisms in Power Distribution
To guarantee automotive systems' safety, dependability, and continuity, fail-safe devices must be incorporated into the power distribution architecture. These systems keep up with necessary operations even in challenging circumstances by identifying and isolating malfunctions. We shall examine these important facets of vehicle power distribution below.
Detecting and Isolating Faults
To guarantee a vehicle's safe and ongoing functioning, problems must be identified early on and isolated. Here's how to make this happen:
Fault Detection
- Sensors: The system is equipped with several sensors to keep an eye on variables including voltage, current, and temperature. An alarm is set off by any departure from regular operations.
- Diagnostic Tools: Sophisticated diagnostic algorithms monitor system performance continually to find abnormalities or irregularities that might point to a possible issue.
- Self-Monitoring Components: A lot of contemporary parts come with built-in self-monitoring features that let them identify and report problems on their own. For instance, in electric vehicle systems, high-power switching circuitry is found in both DC-DC converters and inverters. When this circuitry malfunctions, the module itself detects it and initiates the appropriate action to put the system in a safe mode. For example, it opens a secondary isolation switch to stop the damaged power component's short-circuit current route.
Fault Isolation
- Circuit Breakers and Fuses: These devices, which are carefully positioned throughout the distribution network, cut off the malfunctioning component of the system to stop it from spreading.
- Solid-State Switching Devices: They provide quick and accurate control, making it possible to isolate defects without mechanical wear.
- Isolation Transformers and Optocouplers: By supplying electrical isolation between various system components, these parts help to prevent errors from spreading widely.
These technologies and equipment work together to guarantee that errors are found and isolated with the least possible effect on the system as a whole.
Maintaining Essential Functions During Fault Conditions
It is essential to keep the vehicle's important systems operating even with fault isolation and detection. This calls for careful planning and design:
Redundancy: By creating backup or secondary circuits, one may make sure that crucial operations continue even if the primary channel fails.
Prioritization of Systems: When a fault condition arises, a well-designed power management system may prioritize the most vital systems, such as the safety, braking, and steering systems.
Battery Backup: If the primary power supply is disrupted, in some situations, specialized battery systems can temporarily power necessary components.
Continuous Monitoring and Adaptive Response: Intelligent controllers keep an eye on the system constantly and adjust to changing circumstances by rerouting electricity as needed to keep it operating. For instance, in typical operating conditions, the high-voltage battery supplies the majority of the power to the high-voltage modules in EVs. However, some of these modules, like the high-voltage battery management system, can also use the vehicle's low-voltage battery to maintain essential operations like contactor control and communication, which can help the system reach a safe state, if the high-voltage battery is unavailable.
Power distribution fail-safe systems are essential to the dependable and sturdy operation of contemporary automobiles. Engineers construct systems that can resist the complex and dynamic circumstances experienced on the road via careful planning to retain critical functions and elaborate design that includes enhanced detection and isolation techniques. The rising complexity of cars will continue to push the technical advancement of these systems, especially in the context of electric and autonomous driving, which places an ever-increasing demand on power distribution networks. This section's ideas offer a strong basis for comprehending and creating robust automobile power systems that put performance and safety first.
直接登录
创建新帐号