Introduction to Power Management in Automotive Systems
With the development of more electrified and sophisticated automobiles, power management in the context of automotive systems has emerged as a crucial and intricate area. For engineers and technicians working on contemporary automobiles, a thorough grasp of power management is essential as the automotive industry continues to change.
Definition and Significance of Power Management
In automotive systems, power management pertains to the effective regulation, allocation, and use of electrical power inside a vehicle. To satisfy the needs of several subsystems, including the engine control unit, entertainment, lighting, and advanced driver-assistance systems (ADAS), this entails controlling the production, conversion, and consumption of electrical energy. The significance of power management lies in:
Ensuring Reliability: Power management guarantees that all electronic systems receive a consistent and uninterrupted power supply, which is critical for safety and functioning.
Energy Efficiency: By maximizing energy utilization, it enhances energy efficiency by lowering emissions, improving fuel economy, and increasing the range of electric vehicles (EVs).
Supporting Advanced Features: Power management becomes essential in managing the power requirements of multiple systems without overloading the vehicle's electrical architecture with the addition of more sophisticated electronics and features.
Historical Perspective on Automotive Power Managementt
Over the past century, there has been a substantial evolution in the notion of power management in automotive applications. Here's a quick overview of how it developed:
Early Days: Early automobile engineering focused mostly on mechanical vehicles with few electrical needs. Basic power distribution became necessary with the development of electrical lighting and ignition systems.
Introduction of Alternators: Generators were supplanted by alternators in the middle of the 20th century, which allowed for more sophisticated electrical systems and more effective power conversion.
Advent of Electronic Control Units (ECUs): An increasingly complex power management system was required to handle complex control systems and sensors as a result of the development of microcontrollers and ECUs in the latter half of the 20th century.
Hybrid and Electric Vehicles: The introduction of electric and hybrid cars resulted in the necessity for sophisticated power management systems to regulate the flow of energy between batteries, motors, and other vehicle subsystems.
Connected and Autonomous Vehicles: The complexity of power management has risen due to recent developments in connected and autonomous driving technologies, necessitating sophisticated systems that can adjust to changing operating circumstances.
The topic of automotive power management is constantly developing, mirroring the technical progress of automobiles. Power management has evolved from the basic distribution of power to lighting systems in the first cars to the sophisticated energy management in today's highly electrified and networked vehicles. Power management is now a multidimensional field that supports the functionality, efficiency, and dependability of contemporary automotive systems. Anyone working in automotive engineering or related fields needs to understand its historical evolution and contemporary importance.
Fundamentals of Electrical Power in Vehicles
Automotive power management is a field that requires a fundamental understanding of the flow of electrical power within vehicles. This section examines the sources of electrical power as well as the dynamic needs brought on by the different electrical loads seen in contemporary cars.
Sources of Electrical Power in Vehicles
A vehicle has several electrical power sources, each with distinct functions and needs.
Internal Combustion Engine (ICE) Driven Alternators: Most gasoline or diesel cars have historically generated electricity using a belt-driven alternator that is attached to the engine. When the engine is operating, the alternator's job is to charge the battery and provide electricity to the other car subsystems.
Batteries: When the engine is off or the alternator is not producing enough energy, the car's battery supplies the energy needed to start the engine and run the electrical system.
Regenerative Braking Systems: Regenerative braking systems in hybrid and electric cars store kinetic energy in the battery for future use by converting it back into electrical energy when the vehicle brakes.
Fuel Cells: Hydrogen and oxygen combine chemically to produce energy in fuel cell cars. Electric motors and other vehicle subsystems are powered by this method.
Solar Panels: To augment the power supply, especially for accessory loads like air conditioning or entertainment systems, several contemporary cars are equipped with solar panels.
External Charging: Through charging stations, plug-in hybrids, and all-electric cars may obtain electricity from external electrical networks.
Electrical Load Requirements in Modern Vehicles
The entire amount of electricity that all of the electrical systems and components in a car require is known as the electrical load. With the addition of complex technologies, the electrical load of a contemporary car has grown significantly, necessitating a more comprehensive approach to power management. Low-voltage battery systems in modern cars can deliver 400A to 800A of maximum current to meet peak load demand scenarios. A few examples of high-power, low-voltage system components' current consumptions in contemporary vehicles are included in the list below:
- Power Steering: 5A to 15A
- Exterior Lighting: 10A to 30A/span>
- Air Conditioning (HVAC): 10A to 20A
- Power Windows, Seats: 20A to 30A / motor
- Ignition System: 5A to 15A
- Audio System: 10A to 20A
- Starter Motor: 50A to 150A
The load current applied to the battery might reach hundreds of amperes. Vehicle battery voltage varies with driving conditions. Usually, the loads for 12V systems must continue to function properly throughout the 9V–18V supply voltage range. This criterion also means that the power distribution system must be built so that, under typical operating conditions, each electric load in the system may get a constant supply of at least 9 volts.
Objectives of Power Management
In automobile systems, efficient power management entails more than just delivering energy where it is required. It needs to be carefully balanced and in line with several important goals. These goals are a reflection of the many demands that modern cars must meet, including those related to performance, economy, environmental sustainability, and technological innovation.
Ensuring Reliable Supply of Power
Giving every component of the car a steady and dependable power source is the main goal of power management in automotive systems.
Starting the Vehicle: Even in the worst weather, the combustion engine has to be started with enough power. The cold cranking waveform recommended for low-voltage automotive systems with combustion engines is shown in the following image. In extremely cold weather, cranking increases resistance in both the battery and the harness. As a result, the starting generator's use of current results in a significant drop in battery voltage, reaching as low as 3V, followed by an oscillating waveform that mimics the cranking phenomena that we can also hear from underneath the car at that moment.
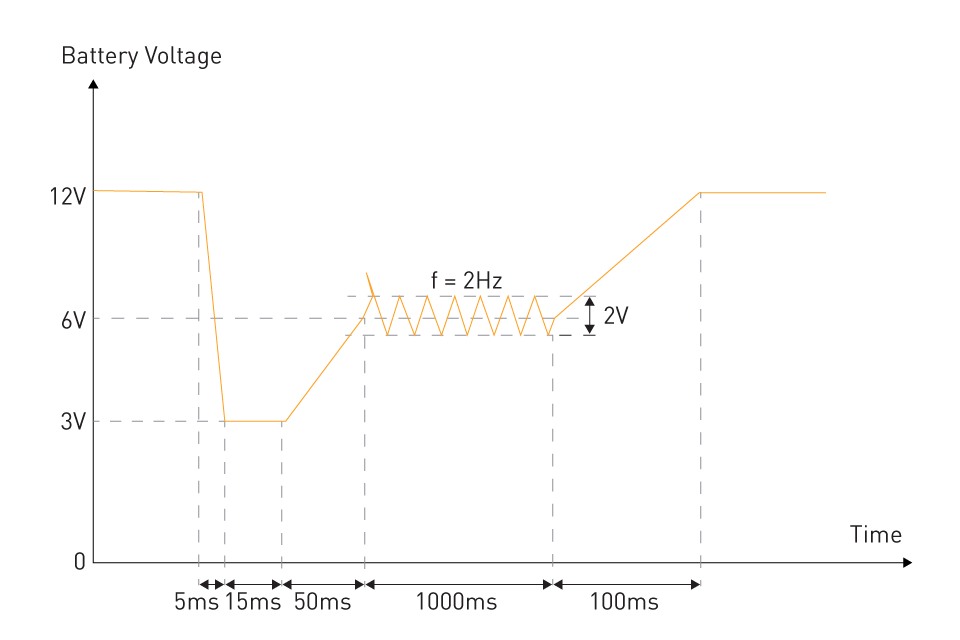
Figure 1: Cold-Cranking Waveform Acc. to ISO 16750-2
Supply During Vehicle Off: Certain systems, such as security systems and some memory operations, need power continuously even while the vehicle is off. When the car is not in use, each load has a budget for quiescent current that it can draw from the battery. This budget, which is usually 100uA per load, ensures that when the car is left alone for an extended period, the battery won't run out too fast.
Supply While Engine is Off: In cars with internal combustion engines, every user is solely dependent on the battery throughout operation while the engine is off. The battery will run out quickly without the alternator. To prevent the battery from draining to a point where it would not be adequate to start the combustion engine, modern cars have battery-saving features.
Managing Power During Peaks and Valleys: Advanced power management makes sure that the traction system delivers the necessary power during peak demands, such as acceleration. For instance, in a HEV car, the combustion engine may start to function in tandem with the e-Machine. The vehicle management system ensures that power consumption is minimized during low generation times (such as idling at a traffic signal) by, among other things, shutting down the combustion engine and deactivating or turning off auxiliary consumers, such as heaters and cooling fans.
Enhancing Fuel Efficiency and Reducing Emissions
To achieve the more general objectives of increasing fuel efficiency and reducing environmental impact, power management is essential.
Optimizing Alternator Operation: Smart alternators used in modern cars are managed by the ECU. Fuel consumption can be reduced by controlling the alternator's activity in accordance with real demand to prevent wasteful energy generation.
Regenerative Braking: Regenerative braking minimizes the need for gasoline or external charge in hybrid and electric cars by transforming braking energy into stored electrical power.
Load Shedding: You may preserve fuel economy by momentarily turning off non-essential systems when load demands are high.
Extending Battery Life
An important goal for electric, hybrid, and conventional automobiles alike is to increase battery life.
Optimal Charging: By avoiding deep draining and overcharging, controlled and intelligent charging may greatly extend battery life.
Energy Balancing: Maintaining the health of a battery pack involves making sure that its cells are charged and discharged equally.
Thermal Management: Extending the life of batteries, particularly in electric cars, requires proper cooling and heating of the batteries. High-power batteries with liquid cooling are a popular remedy.
Accommodating Advanced Electronics and Features
Power management has become increasingly complex due to the powerful electronics and features found in modern automobiles.
Powering Advanced Driver Assistance Systems (ADAS): For these systems to operate correctly, a sizable and steady source of electricity may be needed.
Managing Infotainment Systems: Power management makes sure that information and entertainment systems run smoothly without interfering with necessary car operations. For instance, the entertainment system components may be turned off by the system to preserve these vital car operations if the battery voltage becomes dangerously low.
Adapting to User Customization: Modern cars have a lot of features that consumers may change, such as the lighting and comfort level, which calls for flexible power management techniques.
Beyond only distributing electrical energy, power management in automobile systems aims to achieve much more. They provide an all-encompassing strategy that aims to strike a balance between lifespan, efficiency, dependability, and technological innovation. The tactics used to accomplish these goals must take into account the dynamic and intricate nature of contemporary automobile design and engineering, as well as the constantly shifting circumstances surrounding the vehicle's surroundings and style of operation.
Using creative solutions is necessary for the complex field of automotive power management to match different systems and functions with effectiveness, dependability, and performance. These tactics are a component of a comprehensive plan to maximize the vehicle's power flow and use rather than stand-alone methods. The subsequent sections explore some fundamental concepts that serve as the basis for contemporary vehicle power management systems. While load shedding is the purposeful disabling of non-essential services to preserve electricity and accomplish load management, load management entails dynamically shifting power resources to satisfy the demands of various systems within the vehicle. Prioritization of Systems: Power may be allocated where it is most required by prioritizing safety-critical systems and other systems based on their relative relevance. Monitoring and Control: The electrical system of the car is continuously monitored, enabling real-time modifications to satisfy the needs of different subsystems. Load Shedding in Critical Situations: It is possible to temporarily disable non-essential systems in times of high power demand or low supply to guarantee that important operations have enough power. For instance, in the event of a battery system failure, the system initially begins to turn down non-essential auxiliary loads like the seat warmers, the AC fan, and the audio system in order to save battery SOC for as long as possible. This is because the alternator is unable to charge the battery, which has reached a critical low voltage. These tactics entail seizing and making use of energy that would be thrown away otherwise. Regenerative Braking: Regenerative braking increases overall efficiency in electric and hybrid cars by converting the kinetic energy lost during braking into electrical energy that is stored in the battery. Up to 70% of the vehicle's kinetic energy may be recovered by modern regenerative braking systems. Thermal Energy Harvesting: There are several applications for the surplus heat produced by engines or other parts. As an illustration, heating the cabin air can help cut down on the demand for climate system heaters. The thermoelectric generator (TEG), which can produce power from heat, is another use. For instance, BMW showcased many TEG application prototypes back in 2011, however, there are still issues with mass production and pricing of this component. Solar Energy Harvesting: Adding solar panels to a vehicle's design can offer an extra power source for certain uses, but this is still a difficult option because solar panels are expensive and take up a lot of space on a vehicle. By incorporating intelligence into the process, smart charging solutions go beyond conventional charging methods. Unlike "dumb" charging stations which are limited to communicating solely with the car, smart chargers enable the owner of the charging station to optimize energy use by remotely monitoring, managing, and restricting the use of their devices. Adaptive Charging Algorithms: The charging system may adjust to deliver the best possible charging cycles by recognizing the state of the battery, use trends, and outside variables like temperature. Vehicle-to-Grid (V2G) Integration: By interacting with the power grid, electric cars may replenish energy during periods of high demand and recharge during off-peak hours. The car must have two-way onboard chargers to integrate this feature. On-Board and Remote Diagnostics: By offering comprehensive data on battery health and performance, smart charging solutions enable more effective use and preventative maintenance. Modern cars are complicated and demanding, which is reflected in the growing sophistication of power management systems used in the automobile industry. These tactics provide a comprehensive strategy that takes into account efficiency, dependability, environmental issues, and the rapidly changing technology landscape, rather than just being technical fixes. These strategies, which include the clever integration of charging technologies, the creative capturing of waste energy, and the intelligent allocation of power through load management, represent a dynamic field of ongoing exploration and innovation, providing exciting opportunities for further advancement in the automotive industry. Power Management Strategies
Load Management and Load Shedding
Energy Harvesting and Regenerative Braking
Smart Charging Systems
直接登录
创建新帐号