Introduction to Fuel Injection Systems
Modern internal combustion engines are not complete without fuel injection systems, which offer precise control over fuel supply and are therefore essential for controlling emissions, performance, and economy. The basic ideas, forms, and parts of fuel injection systems are covered in this section.
Basic Principles of Fuel Injection
The carefully regulated procedure of injecting gasoline into the engine's combustion chamber is known as fuel injection. The following are the main ideas behind fuel injection:
Controlled Metering: Optimal combustion and lower waste and emissions are made possible by precise control over the amount of fuel injected.
Spray Atomization: To ensure full combustion and increase efficiency, fuel is sprayed in tiny droplets.
Timing Control: By delivering fuel at the appropriate stage of the engine cycle, injection timing adapts to changes in load and speed.
Types of Fuel Injection Systems
Different fuel injection system designs have been created to accommodate different engine needs.
Direct Injection (DI)
- Fuel is injected directly into the combustion chamber.
- Precise control and efficient combustion is enabled, especially at high loads.
Port Injection (PI)
- Fuel is injected into the intake manifold or port using port injection (PI). It is frequently utilized in passenger autos and offers excellent mixture preparation.
Sequential Fuel Injection (SFI)
- Enables each injector to be independently controlled while synchronizing with the engine's firing sequence.
- Performs better by giving you more exact control over the fuel flow to each cylinder.
Throttle Body Injection (TBI)
- Injects fuel into the throttle body, which is located upstream of the intake manifold.
- Generally regarded as a more straightforward technology than the others.
Components of Fuel Injection Systems
Fuel injection systems are made up of many essential parts:
Injectors: Accurate tools that precisely dispense fuel into the engine. There are several designs available for different uses.
Fuel Rails: These are the pipes that supply fuel to the injectors while preserving the required pressure.
Fuel Pump: They are used to transfer fuel from tanks to fuel rails; they frequently come along with pressure regulators to regulate fuel pressure.
Engine Control Unit (ECU): The engine control unit (ECU) is the brain of the system; it uses sensor inputs to determine the necessary amount of fuel and the timing of injections.
Sensors: The ECU receives real-time data from a variety of sensors, such as mass airflow and oxygen sensors, to enable adaptive control.
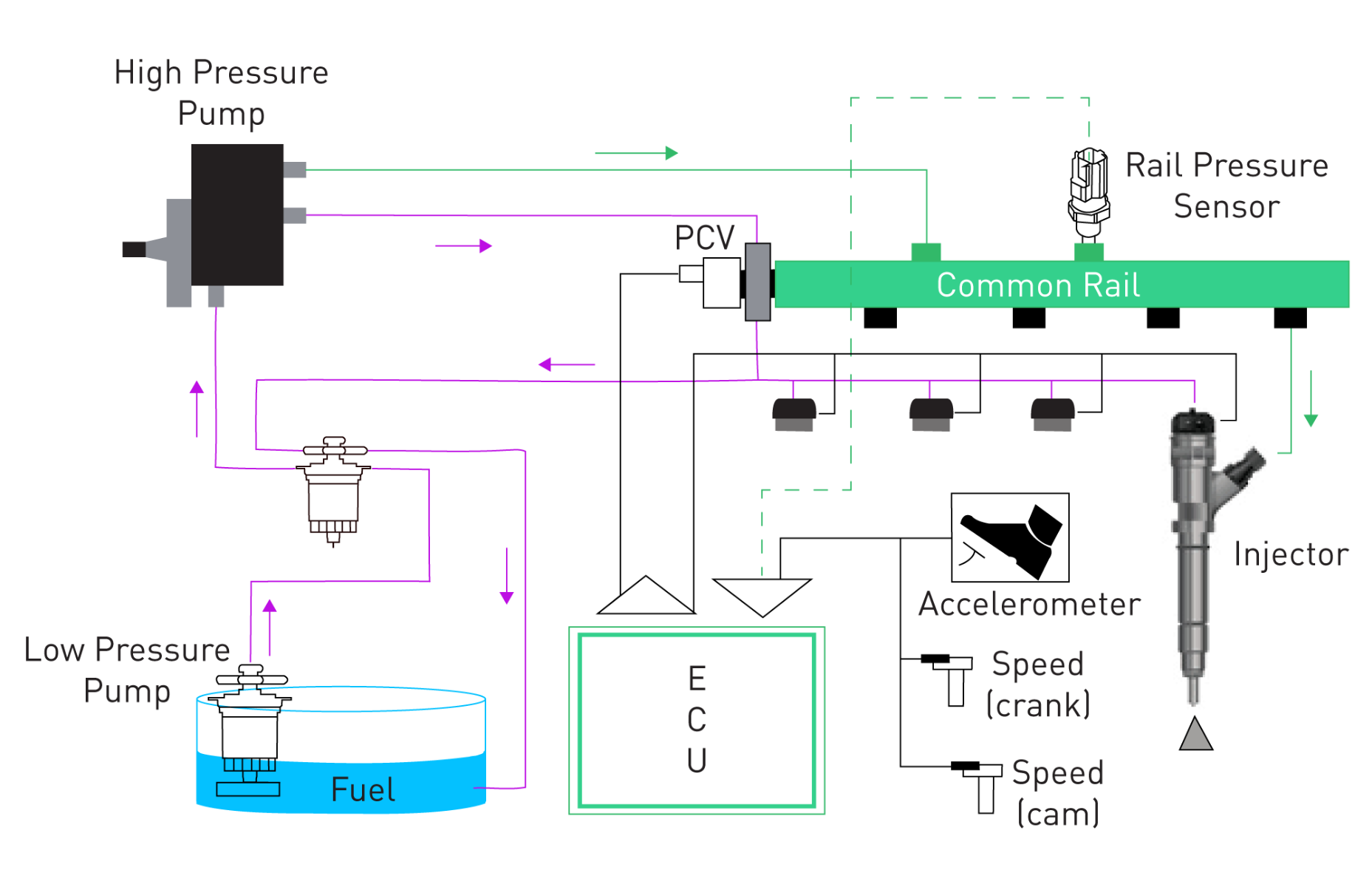
Figure 1: Depiction of a Typical Fuel Injection System
In many applications, fuel injection systems have taken the place of carburetors as a fundamental component of contemporary internal combustion engines. Fuel injection systems provide increased responsiveness and efficiency using highly regulated atomization, timing, and metering. Fuel injection may be customized to work with a broad range of engine types and operating situations thanks to the variety of kinds and components that allow for design freedom. Gaining an understanding of the fundamentals and elements of fuel injection systems paves the way for investigating the integrations and control schemes that drive contemporary engine management.
Fuel Injection Control Strategies
Modern internal combustion engines depend on accurate fuel injection regulation to function, which affects efficiency, emissions, and performance. The primary control schemes for fuel injection systems will be discussed here, with an emphasis on air-fuel ratio management, timing and duration control of injections, and adaptive controls for changing engine and environmental circumstances.
Air-Fuel Ratio Control
The amount of fuel to air in the engine's intake mixture is known as the air-fuel ratio, or AFR. To preserve fuel efficiency, minimize emissions, and achieve efficient combustion, precise control of AFR is essential. Important elements consist of:
Stoichiometric AFR: This is the optimal ratio, which for gasoline is typically about 14.7:1 when all of the fuel is utilized with the available oxygen. In many driving situations, it is a target and reduces hazardous emissions.
Lean and Rich Mixtures: Higher AFR lean mixes can boost fuel economy, but there is a danger of increased NOx emissions and engine knocking. To boost power, rich mixes (lower AFR) may be utilized, however, this will result in worse fuel efficiency and more CO emissions.
Lambda Control: Oxygen sensors, also known as lambda sensors, are used to detect the amount of oxygen in exhaust. They then provide the ECU with input so that the AFR may be adjusted appropriately.
Closed-Loop Control: A feedback control system that continually modifies the injector pulse width while taking temperature, load, and other variables into account in order to maintain the intended AFR.
Injection Timing and Duration Control
For optimal engine performance and economy, fuel injection timing and duration are also critical factors.
Injection Timing: When the fuel injection begins in relation to the engine's cycle, is referred to as injection timing. It might occur before, during, or after the intake stroke, based on the kind of engine and the circumstances of operation.
Injection Duration: This indicates how long the injector is left open, which affects how much fuel is supplied. The throttle position, engine speed, and load are some of the variables that the ECU uses to determine the necessary time.
Multiple Injections: With the ability to carry out several injections in a single cycle, modern systems offer more exact control over emissions, noise, and combustion.
Adaptive Controls for Varying Engine and Environmental Conditions
A broad range of operating variables, including variations in load, temperature, altitude, and fuel quality, must be accommodated by modern engines. Important coping mechanisms include:
Load Sensing: This ensures efficient engine performance at both idle and maximum power by adjusting the fuel injection to meet the engine's load.
Temperature Compensation: While hot engines can need tweaks to stop banging, cold engines need a richer mixture.
Altitude Compensation: Since there is less oxygen in the air at higher elevations, the AFR must be adjusted.
Fuel Quality Sensing: Engines may adjust the injection method based on the properties of different fuels.
Modern engine management systems are based on fuel injection control techniques, which provide accurate combustion control to fulfill strict pollution regulations and performance goals. Modern fuel injection systems contribute to the intricate orchestration needed to run the wide variety of internal combustion engines that are available today by dynamically altering the air-fuel ratio, injection timing, and reacting to different engine and environmental circumstances. Fuel injection control is an area of constant innovation and optimization because of the advancement of sensors, algorithms, and hardware.
Introduction to Ignition Systems
An essential component of internal combustion engines, ignition systems light the air-fuel combination, starting the combustion process. A well-thought-out ignition system has to provide a spark with sufficient energy and timing to guarantee thorough and effective combustion. The fundamentals of ignition and the many kinds of ignition systems frequently used in automobile engines are covered in this chapter's section.
Basic Principles of Ignition
The compressed air-fuel combination in the engine's cylinder is ignited by a spark, which starts the ignition process and causes quick combustion and energy release. Among the fundamental ideas are:
Spark Creation: A spark is produced by passing a high-voltage electric current between the electrodes of a spark plug. An ignition coil provides the energy for this by converting the low-voltage electrical supply of the car into the necessary high-voltage.
Ignition Timing: The timing of the spark is crucial to the engine's operation. Typically, it happens shortly before the piston hits the Top Dead Center (TDC) during the compression stroke. For the best engine performance, fuel efficiency, and emissions management, timing is essential.
Energy Transfer: The spark must have sufficient energy to ignite the mixture, but not so much that it gradually erodes the spark plug electrodes or other parts.
Types of Ignition Systems
Diverse ignition system types have been created to accommodate different engine needs and technology developments. Among the primary kinds are:
Distributor Ignition System: In this conventional setup, high voltage is sent to the appropriate spark plug at the appropriate moment using a distributor that is mechanically operated. Timing changes are frequently performed mechanically, or by using vacuum and electrical controllers.
Distributorless Ignition System (DIS): This system uses separate coils for one or more cylinders, eliminating the need for a distributor. To adjust spark timing, it frequently uses a computer-controlled module that collects inputs from sensors.
Coil-on-Plug (COP) Ignition System: With this more recent method, each spark plug has a single ignition coil immediately on top of it, providing more precise control and increased energy efficiency. The COP system eliminates energy loss and improves ignition dependability by shortening the spark's distance of travel.
Wasted Spark System: This system uses two cylinders in pairs to fire two spark plugs concurrently using one coil. One spark is "wasted" in an exhaust stroke cylinder, while the other ignites the mixture in its cylinder. Though less effective than COP, it is easier.
From distributor systems that were mechanically controlled to intricate electronically controlled designs, ignition systems have developed throughout time. The objective is still the same: to ignite the air-fuel mixture with a prompt, powerful spark. Automotive engineers must optimize ignition systems for cost, performance, emissions, and dependability, thus they must have a thorough understanding of the fundamentals and variants of these systems. The drive in automobile design towards more control, efficiency, and environmental responsibility is reflected in the tendency toward more accurate and efficient systems, such as the Coil-on-Plug.
Ignition System Control Strategies
With the development of the engine control unit (ECU), where exact management methods are necessary for maximum engine performance, the current ignition system has become a highly complicated component. Important tactics include knock detection and management, ignition timing control, and spark advance and retard schemes. The importance of these techniques in automobile engineering is discussed in more detail in this section.
Ignition Timing Control
Definition and Importance: The exact instant the spark plug should ignite, in order to ignite the air-fuel combination within the cylinder is referred to as ignition timing. To maximize power, reduce emissions, and maximize fuel efficiency, this timing must be properly controlled.
Static vs. Dynamic Timing
- Static Timing: A predetermined ignition point that ignores load or engine speed.
- Dynamic Timing: Modifies ignition timing based on several engine parameters, including speed, load, and temperature.
Control Methods
- Mechanical: The distributor uses springs and centrifugal weights.
- Electronic: For more precise control, modern systems make use of sensors and the ECU.
Challenges
Ignition timing management may be difficult to implement when competing needs like performance, efficiency, and emissions need to be balanced.
Spark Advance and Retard Strategies
Spark Advance
- Definition: Earlier ignition of the air-fuel combination during the compression stroke of the piston is achieved by advancing the spark.
- Purpose: Useful at high speeds or with heavy loads to give the combustion process more time.
- Effect: May boost engine power, however, excessive usage may cause engine knocking.
Spark Retard
- Definition: Delaying the spark causes the mixture to ignite later in the compression stroke.
- Purpose: Useful in low-load situations or when knock is felt.
- Effect: Can cut electricity, but also lessens emissions and the chance of knocking.
- Control Strategy: Based on a variety of sensor inputs, modern engines continually modify the spark timing, advancing or retarding as necessary.
Knock Detection and Control
Engine Knock: A premature and uncontrollably burning combination of air and gasoline that can harm an engine.
Knock Sensors: It uses piezoelectric sensors to identify knock induced vibrations.
Control Strategies:
- Spark Retard: As previously indicated, spark retardation can aid in preventing knock.
- Fuel Mixture Adjustment: Cooling the combustion process and avoiding knock may also be achieved by enriching the air-fuel mixture.
Importance of Control: Well-executed knock control protects the engine from any harm while optimizing performance and fuel economy.
A crucial component of contemporary engine management is the use of ignition system control techniques. Engineers may maximize pollution control, fuel efficiency, and engine performance by comprehending and putting these techniques into practice. These techniques are essential for the development of automotive technology because of the rising demand for ecologically friendly automobiles. Students who pursue further education in this discipline will be better prepared to innovate and make valuable contributions to the dynamic field of automotive engineering.
Integrating Fuel Injection and Ignition Controls
Modern internal combustion engines must integrate their fuel injection and ignition control systems in order to provide the best possible performance, efficiency, and emissions control. This part explores the intricacies and innovations of this integration, emphasizing coordinated control for peak performance and going over the problems and solutions that come with it.
Coordinated Control for Optimal Performance
Interdependence of Fuel and Ignition: To guarantee ideal combustion, the timing of the ignition spark and fuel injection must be precisely synchronized. Variations in engine speed, load, temperature, and other factors must be handled by both systems.
Control Objectives
The goal of coordinated control is:
- Efficiency: To maximize fuel conversion to energy.
- Emissions Control: Ensuring full combustion to reduce hazardous emissions.
- Performance: Improving the properties of power and torque.
Techniques
- Synchronized Mapping: The Engine Control Unit (ECU) employs pre-established maps that link spark timing and fuel injection for various engine circumstances.
- Adaptive Control: This refers to the ability of learning algorithms to instantly adapt to variations in fuel quality, environmental factors, or wear and tear.
Benefits
- Fuel Economy: Enhanced fuel efficiency may be achieved with harmonized management.
- Responsive Performance: The integration guarantees faster reactions to inputs from the driver, such as braking and acceleration.
- Longevity: Engine components are not so stressed by balanced combustion.
Challenges and Solutions in Integration
Challenges
- Complexity: The sheer quantity of variables to take into account, together with their interconnectedness, contributes to the complexity.
- Compatibility: There may be intrinsic variations between various fuel injection and ignition system types that require attention.
- Real-Time Requirements: In milliseconds, the system must process massive volumes of data and make judgments.
- Cost: The expense of sophisticated controls is increased by the need for sophisticated hardware and software.
Solutions
- Advanced Algorithms: Making use of multidimensional input processing methods and contemporary control theory.
- Simulation and Modeling: Creating reliable controllers requires extensive testing via simulation.
- Standardization: Interoperability is ensured by establishing common standards for various components.
- Hardware Acceleration: Needs for real-time calculation can be met by specialized processors.
A key component of contemporary engine technology is the integration of fuel injection and ignition controls, which significantly improves engine performance, efficiency, and compliance with environmental standards. Although there are many engineering hurdles associated with this integration, substantial advancements have been made possible by breakthroughs in hardware, algorithms, and standards.
For both professionals and engineering students, comprehending this connection will be crucial as the automobile industry develops further. It highlights how multidisciplinary automotive engineering is and how knowledge in fluid mechanics, thermodynamics, control systems, and other fields is necessary. This subject is a fascinating one for further innovation and study as future improvements may involve more integration with other subsystems like exhaust after-treatment and hybrid powertrain controls.
直接登录
创建新帐号