Power Conversion in Electric Vehicles
Effective power conversion is essential to achieving maximum performance, longevity, and range in electric vehicles (EVs). The vehicle's subsystems are powered by converting the energy stored in the batteries into forms that can be used. Key power conversion technologies are covered in detail in this section of the chapter, with special attention to DC-DC converters, onboard chargers, and DC fast charging systems.
DC/DC Converters
Functionality: To power headlights, interior lights, wiper and window motors, fans, pumps, and many other low-voltage systems in electric vehicles (EVs) and hybrid electric vehicles (HEVs), DC-DC converters convert DC power from higher voltage batteries into lower DC voltages (48V or 12V).
Efficiency Considerations: These devices use modern low-loss switching devices like SiC MOSFETs along with busbars for power transfer because choosing and designing the correct converter is essential to ensuring that as little energy is lost during the conversion process, which directly affects the vehicle's overall energy efficiency.
Onboard Chargers
Functionality: For the purpose of charging the car's battery, onboard chargers manage the transformation of grid-supplied AC power into DC power. Every HEV and plug-in EV depends on them.
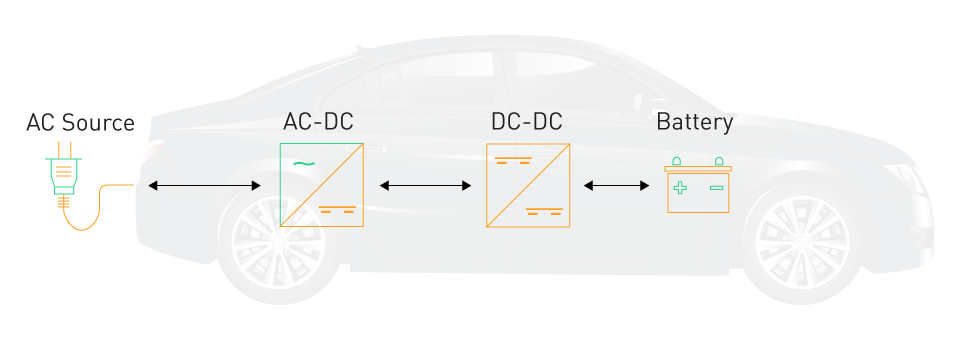
Figure 1: Onboard Charger Block Level Diagram
They are split up into two primary groups. Using a PFC (Power Factor Correction) circuit, the first block ensures that the mains AC voltage is converted to DC voltage. Converting the DC voltage to the voltage level, the battery needs is in the second stage. For instance, a PFC Boost Converter is frequently incorporated into the first block to increase the AC voltage to approximately 400V DC voltage from 220VAC Mains Voltage. This voltage may be raised to the 800V battery level by the second stage.
Charging Levels: These chargers may operate at different levels, typically Level 1 (120 VAC) and Level 2 (240 VAC), affecting the charging speed.
Communication and Control: Smart charging strategies and user-friendly interfaces are made possible by the communication features that modern onboard chargers frequently include to interact with charging stations.
Efficiency and Compatibility: It is a design challenge to ensure high conversion efficiency and compatibility with different charging infrastructures.
DC Fast Charging
Functionality: The vehicle's battery receives direct current (DC) power from DC fast charging stations at a significantly faster rate than from conventional onboard chargers. With this, charging times can be drastically lowered to as little as 15 minutes, allowing for rapid charging.
Standards and Protocols: Connectors, communication, and other aspects are defined by a number of standards for DC fast charging, including CHAdeMO and CCS (Combined Charging System).
Infrastructure Requirements: Strong grid support and cutting-edge charging hardware are required for the implementation of DC fast charging. Public charging networks must take this into account.
Vehicle Compatibility: The high power levels offered by DC fast chargers are not compatible with all EVs. The technology of batteries, thermal control, and vehicle electronics must all be carefully considered.
The performance and user experience of electric vehicles are directly impacted by the complex but crucial field of power conversion. It is imperative that engineers, policymakers, and stakeholders in the automotive and energy sectors comprehend the functions and nuances of DC-DC converters, onboard chargers, and DC fast charging.
These technologies affect grid management, energy consumption, and the spread of electric vehicles (EVs) across multiple markets in addition to defining the end-users' experience with charging.
The shift to efficient and sustainable electric transportation systems will be largely determined by ongoing innovation in power conversion technologies combined with clever energy management techniques.
Energy Management Strategies
Optimizing the efficiency, range, and performance of electric vehicles requires effective energy management. This entails a number of techniques and innovations that control how energy is held, used, and recovered by the car’s systems. This section will examine two core components of energy management: regenerative braking systems and energy storage systems, such as batteries and super-capacitors.
Energy Storage Systems (Batteries and Supercapacitors)
Batteries
Functionality: In electric vehicles (EVs), batteries serve as the main energy storage component, storing electrical energy for driving and other vehicle operations.
Types: The majority of EVs run on lithium-ion batteries, while some also employ Nickel-Metal Hydride batteries, which have a lower energy density.
Management: The state of charge, temperature, current, and other characteristics are monitored and controlled by Battery Management Systems (BMS) to guarantee optimal battery performance.
Challenges and Opportunities: Energy density, weight, longevity, and cost are design factors. The development of EV capabilities is largely dependent on advances in battery technology.
Supercapacitors
Functionality: Super-capacitors are capable of fast charging and discharging and storing energy via electrostatic methods. In certain EVs, they work in tandem with batteries.
Applications: Because super-capacitors can swiftly absorb regenerated energy, they are especially helpful for short-term energy storage in systems like regenerative braking.
Advantages and Limitations: Super-capacitors have a substantially lower energy density than batteries while having a high power density and quick charging times.
Regenerative Braking Systems
Principles: Regenerative braking is a technique that recovers and recycles the kinetic energy of a moving vehicle, which is typically lost as heat when braking. This energy is repurposed as electrical energy rather than being wasted.
Functionality: In order to transform the kinetic energy of the car into electrical energy that is stored in the battery or super-capacitors, the e-Machine runs in reverse and functions as a generator when driving.
Integration with Conventional Braking: The coordination of regenerative braking and traditional friction brakes is a crucial design consideration because they are frequently used in tandem.
Efficiency and Performance: Driving conditions affect how effective regenerative braking is. When implemented properly, total vehicle efficiency can be greatly increased. Regenerative braking is generally 60–70% efficient.
Challenges: The main challenges are ensuring a seamless transition between regenerative and friction braking, controlling the quick charging of energy storage, and being compatible with vehicle dynamics.
EVs have complex energy management systems that are essential to the vehicle's overall effectiveness and performance. Modern electric vehicle design revolves around these factors, which include the sophisticated chemistry and physics of batteries, the quick response of super-capacitors, and the clever blending of regenerative and conventional braking.
Further developments in these fields should improve the EVs' affordability, sustainability, and range, which will help them become more and more common in the world's transportation system. For the production of vehicles that meet the demanding requirements of modern mobility while minimizing environmental impact, engineers, manufacturers, and policymakers must collaborate and utilize these strategies.
Power Control and Optimization
In order to maximize performance, increase energy efficiency, extend range, and improve the driving experience overall, an electric vehicle's (EV) power flow must be efficiently controlled. Achieving these goals largely depends on the use of specialized controllers in conjunction with intelligent control strategies. The two main topics of power control and optimization in EVs covered in this section are energy management controllers and control strategies for power train optimization.
Control Strategies for Powertrain Optimization
An electric vehicle's power train consists of its gearbox, e-machine, energy storage devices (like batteries), and related electronic controllers. A multi-faceted strategy is needed for power train optimization:
Energy Efficiency: Implementing energy-saving measures into practice by utilizing regenerative braking, controlling battery usage, and improving motor control.
Performance Tuning: Modifying control parameters in accordance with driver preferences and driving circumstances to improve acceleration, top speed, and responsiveness.
Thermal Management: Temperature control of vital parts, like the motor and battery, to guarantee longevity and safety.
Integration with Ancillary Systems: Compatible operation of the vehicle’s climate control and stability control systems can be achieved by coordinating power train control with them.
Adaptation to Driving Conditions: Putting into practice adaptive algorithms that adapt to different driving conditions, such as traffic, weather, and terrain, in order to maximize effectiveness.
Energy Management Controllers
The distribution and flow of energy inside the car are controlled by Energy Management Controllers (EMCs), which are specialist hardware and software systems. To achieve the best possible power control, they are essential in:
Functionality: Energy management controllers (EMCs) regulate the energy transfer between the battery, motor, auxiliary systems, and regenerative braking, guaranteeing optimal energy utilization.
Control Algorithms: There are several control algorithms in use, each with specific benefits and uses, including rule-based logic, fuzzy logic, and model predictive control.
Real-Time Decision Making: Robustness and responsiveness are critical because EMCs have to react quickly to changing conditions, like abrupt braking or acceleration, and must make decisions in real-time.
Integration with BMS: EMCs and Battery Management Systems (BMS) frequently collaborate to maximize battery performance by taking temperature and state-of-charge (SOC) into account.
Communication with Other Controllers: The infotainment system and traction control system, for example, are two controllers in the car that EMCs interact with to provide a seamless driving experience.
Data Collection and Analysis: Personalized driving profiles, predictive maintenance, and continuous improvement are made possible by the data collection and analysis capabilities of modern EMCs.
In EVs, power control and optimization involve a complex interplay of controllers with specialized functions, algorithms, and strategies. Manufacturers of electric vehicles (EVs) are able to meet and even surpass the performance and efficiency requirements of the modern automotive landscape by optimizing powertrain operations and utilizing intelligent energy management controllers.
EVs' capabilities will only grow stronger as more sophisticated control schemes and powerful controllers are continuously developed, securing their position in the transportation industry. This field of engineering requires its practitioners to not only comprehend these complex systems but also to come up with novel approaches to utilizing technology to increase productivity, sustainability, and efficiency.
Grid Integration and Vehicle-to-Grid (V2G) Systems
The increasing popularity of electric vehicles (EVs) has created both new opportunities and challenges for their integration with the electrical grid. In addition to charging the cars, EVs and the grid interact through cutting-edge technologies like Vehicle-to-Grid (V2G) and smart charging. This section will examine the basic ideas behind grid integration and delve into the exciting realm of smart charging and vehicle-to-grid technologies.
Basic Concepts of Grid Integration
Charging Infrastructure: This covers the grid connection of the network of charging stations. There are two types of charging levels (Level 1 and Level 2), each with a different speed and complexity.
Grid Load Management: Integration of EVs introduces additional load to the electric grid. Efficient scheduling and demand-side management are essential to prevent overloading and to balance supply and demand. There are different approaches to load management. With Equally distributed load sharing, each attached vehicle receives the same charge current, 20 amperes in the below example. With first-in load sharing, the first vehicles plugged into the charging network get full charging of 40 amperes. If the electrical panel can provide up to 80 amperes, it means, the third and fourth vehicle has to wait until the first or second vehicles finished charging.
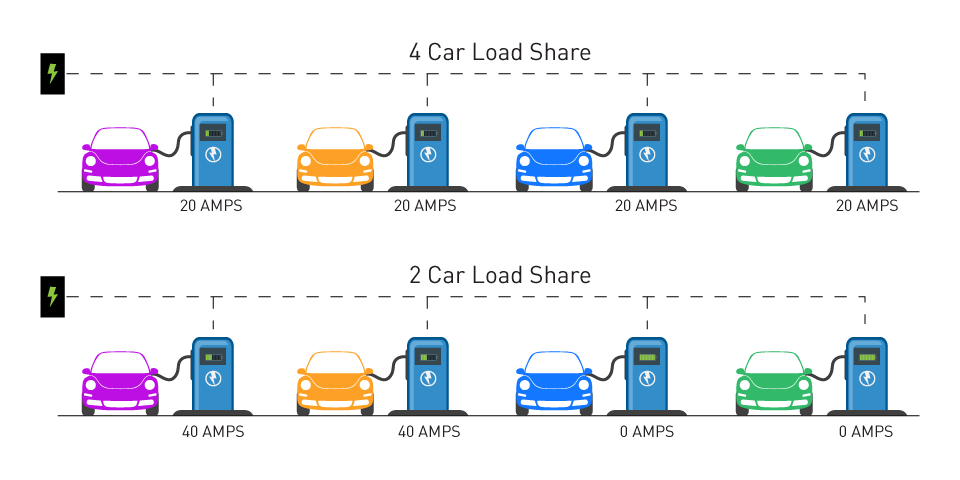
Figure 2: Example of Different Load-Sharing Approaches
Renewable Energy Integration: Integrating EV charging with solar or wind power requires careful coordination to maximize clean energy utilization, especially with the rise in renewable energy sources.
Regulatory Compliance: It is essential to make sure that all grid interactions adhere to legal requirements, such as safety guidelines and energy market laws.
Vehicle-to-Grid (V2G) and Smart Charging Technologies
Vehicle-to-Grid (V2G)
- Definition: Vehicle-to-grid (V2G) technology enables both vehicle charging and vehicle discharging, potentially enhancing grid stability and facilitating the integration of renewable energy sources.
- Grid Support: EVs may feed energy back into the grid during periods of high demand, which aids in frequency management and load balancing.
- Economic Benefits: Owners who supply the grid with electricity or auxiliary services may be paid.
- Challenges: There are technical and legal obstacles that need to be overcome, such as battery warranty concerns and bi-directional charging requirements.
Smart Charging
- Definition: Smart charging optimizes charging depending on a range of criteria, including grid conditions, price, and user preferences. It entails sophisticated, two-way communication between the car, charging station, and grid.
- Demand Response: Smart charging aids in demand-side management by modifying charging schedules and prices in response to grid circumstances.
- Integration with Renewable Sources: It may synchronize charging with the availability of renewable energy, encouraging the use of green energy.
- User Customization: Users may customize their experience by setting choices such as pricing thresholds and charge times.
An important area of development for electric vehicle technology is grid integration and vehicle-to-grid technologies. These technologies provide a means of achieving a more sustainable, resilient, and efficient energy landscape by bridging the gap between individual transportation needs and the collective demands of energy infrastructure.
In order for these programs to be successful, legislators, utility companies, automakers, and consumers must work together. It demands innovation in technology, vision in regulations, and a common dedication to the more general objectives of environmental stewardship and energy sustainability.
Engineers, urban planners, and energy experts will need to comprehend and put these ideas into practice as we head towards a future where EV adoption will increase. An abundance of exciting opportunities for energy management, cost savings, and environmental conservation arise from the synergistic interaction between EVs and the grid.
Case Studies: Implementing Power Conversion and Energy Management in EVs
Power conversion systems and energy management are two areas of constant innovation in the dynamic field of electric vehicle (EV) technology advancement. This section presents a few relevant case studies that shed light on how these technologies are actually used in real-world scenarios.
Power Conversion Systems in Commercial EVs
Tesla Supercharger Network
- Overview: The Tesla Supercharger network is a prime example of cutting-edge DC Fast Charging technology, which dramatically cuts down on how long it takes to charge a Tesla vehicle.
- Technology: To enable quick energy transmission, a special mix of liquid-cooled cables and 480V direct current (DC) charging is used.
- Impact: Has made it possible for EVs to travel vast distances, changing how consumers see electric transportation.
BYD's In-House Power Conversion
- Overview: BYD, a Chinese automaker, achieved a breakthrough with its silicon carbide (SiC) power module, which will be used in its new energy vehicles (NEVs).
- Technology: By using silicon carbide (SiC) components, efficiency may be increased while weight and size are decreased.
- Impact: A move in the direction of EV component integration and cost reduction, increasing accessibility.
Innovations in Energy Management for Electric Vehicles
Nissan Leaf’s e-Pedal and Regenerative Braking
- Overview: The Nissan Leaf is the vehicle that launched the e-Pedal, a mechanism that combines braking, accelerating, and deceleration into one pedal.
- Technology: To save energy, it uses clever energy management and regenerative braking.
- Impact: Optimizes energy recovery and improves driving convenience.
BMW's Intelligent Energy Management
- Overview: BMW's electric and hybrid cars are equipped with an extensive energy management system.
- Technology: To ensure maximum efficiency, energy flow between the battery, e-Machine, and auxiliary components is controlled using clever algorithms.
- Impact: Enhances system performance and driving range.
Volvo Buses - Energy Storage with Supercapacitors
- Overview: Supercapacitors are used by Volvo to store and release energy quickly.
- Technology: Supercapacitors reduce battery burden by storing energy during braking and supplying it during acceleration.
- Impact: Increases effectiveness and prolongs battery life.
These case studies show how cutting-edge approaches to energy management and power conversion are reshaping the EV market. Electric mobility is changing as a result of the integration of advanced technologies, from individual consumer cars to large commercial fleets.
Policymakers, industry leaders, and engineers can gain valuable insights from the lessons learned and the successes of these implementations. They place a strong emphasis on the value of ongoing research, development, teamwork, and technology adoption.
These examples demonstrate what can be accomplished in the field of electric transportation in a world where efficiency, sustainability, and innovation are extremely important. The future of electric mobility will undoubtedly remain an exciting and quickly developing field thanks to the continuous search for improvements in these areas.
直接登录
创建新帐号