Introduction to Delta-Sigma ADCs
Delta-Sigma (∆Σ) ADCs, also referred to as Sigma-Delta ADCs, occupy a distinctive niche in the spectrum of analog-to-digital converters. These ADCs are widely known for their exceptional precision and accuracy, finding prominence in applications where precision holds paramount significance, such as audio processing and instrumentation.
At the core of the Delta-Sigma ADC lies the paradigm of oversampling, synergized with noise shaping and digital filtering. The essence of oversampling entails sampling the analog input at a significantly higher rate than the Nyquist rate. This oversampling doesn't aim to enhance signal granularity, but rather to disperse quantization noise across a broader frequency spectrum. This extended noise dispersion is subsequently shaped, ensuring a substantial portion lies beyond the frequency scope of interest. Following digital filtering (decimation), the sample rate is reduced while out-of-band noise is removed.
Delta-Sigma ADCs strike a balance by exchanging a heightened sampling frequency for augmented resolution within the designated frequency range of interest.
Delta-Sigma Modulator: Central to the architecture of the Delta-Sigma ADC is the Delta-Sigma modulator. This modulator hinges on a negative feedback loop comprising an integrator, a comparator, and a 1-bit digital-to-analog converter (DAC). The integrator accumulates the discrepancies between the input signal and the 1-bit DAC's output. Subsequently, the comparator scrutinizes this integrated output against a reference voltage. The outcome materializes as a 1-bit data stream, which is then fed back via the 1-bit DAC, orchestrating the iterative feedback loop.
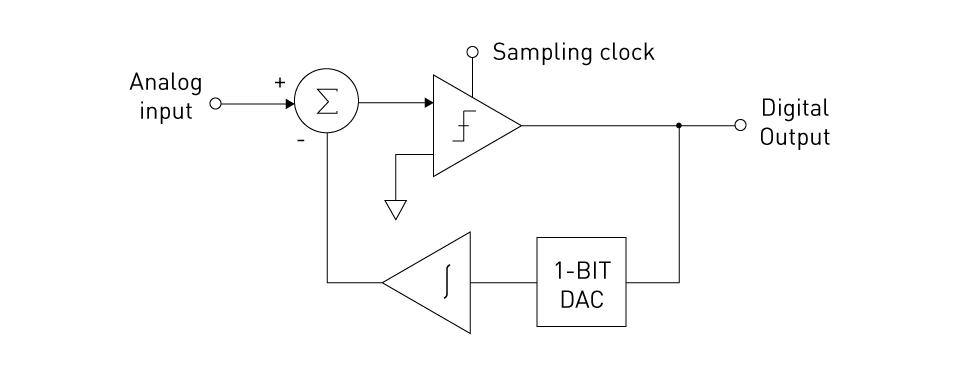
Figure 5: Delta modulator
Decimation Filter: Following the Delta-Sigma modulator, the 1-bit data stream is subjected to decimation through a digital filter. This operation encompasses data averaging and the reduction of the sample rate, resulting in a multi-bit digital output. The process of decimation serves to concentrate the pertinent information of the signal into a narrower bandwidth, thereby elevating resolution while tempering quantization noise within the designated band of interest.
In its essence, the Delta-Sigma ADC embodies a harmonious fusion of analog and digital processing. Its proficiency in achieving high-resolution conversion is realized through the orchestration of oversampling, noise shaping, and decimation, deftly navigating the intricate balance between swiftness and precision.
Architecture of Delta-Sigma ADCs
The integrator, 1-bit DAC, comparator, and decimation filter are the four major components to examine.
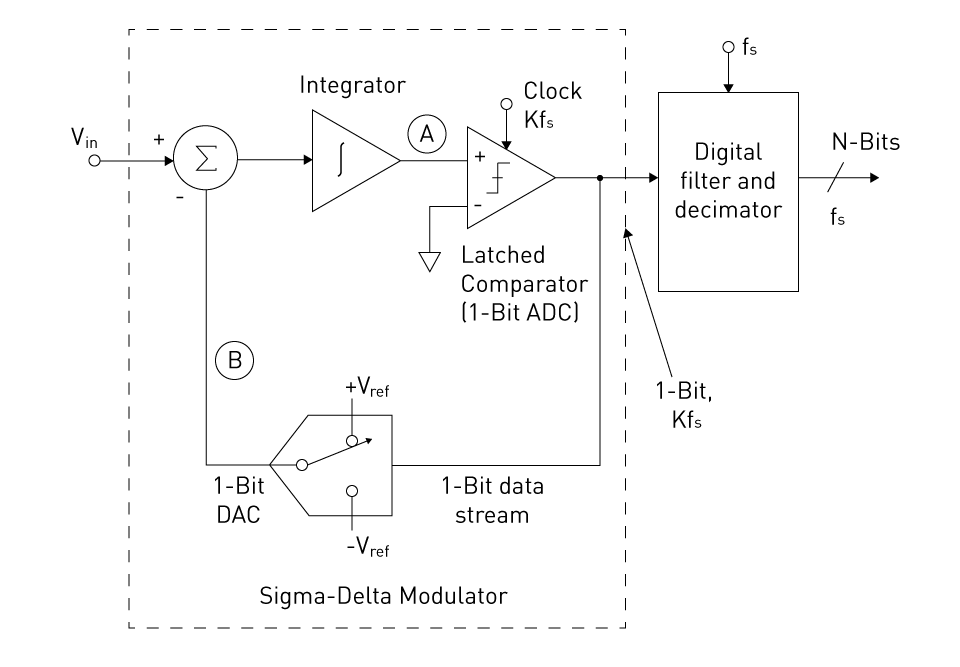
Figure 6: First-order Sigma-Delta ADC
Integrator
Functioning as stage one of the Delta-Sigma ADC, the integrator assumes a fundamental role. It embraces the incoming analog signal alongside the output emanating from the 1-bit DAC, meticulously computing the integral of the disparity between these two signals. This pivotal operation lays the groundwork for the error feedback mechanism, an indispensable cornerstone of the Delta-Sigma ADC's operational framework. In addition, the integrator introduces a deliberate attenuation in the signal's rate of change of the signal, consequently augmenting the converter's resolution during instances of lower frequencies.
1-bit DAC
At the heart of the feedback loop lies the 1-bit Digital-to-Analog Converter (DAC). It receives the digital output generated by the comparator and transforms it back into an analog waveform. This reconstituted signal is subsequently juxtaposed against the original input within the integrator, thereby orchestrating the conversion of quantization error into a form of negative feedback. The Delta-Sigma ADC charts its trajectory of high-resolution performance by perpetually rectifying errors through this mechanism.
Comparator
Employed as a 1-bit quantizer, the comparator fulfills the role of generating a binary representation derived from the integrator's output. It accomplishes this by juxtaposing the integrator's output against a predetermined reference voltage, often set at zero for a 1-bit comparator. By gauging the sign of the resultant disparity, the comparator issues a digital output indicative of the observed difference.
Decimation Filter
As the concluding phase of the Delta-Sigma ADC's operation, the decimation filter takes center stage. This digital low-pass filter serves a dual purpose, encompassing noise shaping and rate reduction. Operating as the ADC's final arbiter, the filter addresses two pivotal tasks. It curbs the out-of-band quantization noise stemming from the Delta-Sigma modulator, orchestrating its attenuation while emphasizing higher frequencies. Additionally, the decimation filter mitigates the oversampled data rate, transforming it into a more manageable pace. This process is called decimation, and it engenders an augmented signal resolution within the confines of the frequency band under scrutiny.
Finally, the architecture of a Delta-Sigma ADC converts an analog input signal into a high-resolution digital output via the smart combination of the integrator, 1-bit DAC, comparator, and decimation filter. Each component is critical in ensuring that Delta-Sigma ADCs deliver outstanding accuracy, especially in low-frequency and high-resolution applications.
Operation of Delta-Sigma ADCs
Delta-Sigma ADCs work by combining three fundamental techniques: oversampling, noise shaping, and decimation. These strategies enable the ADC to transform an analog input signal with high resolution and low noise into a digital output signal.
Oversampling
Oversampling entails the act of sampling an input signal at a notably higher frequency than the Nyquist rate, which is precisely twice the signal bandwidth or the highest frequency within the baseband signal. Oversampling brings about a significant advantage by simplifying the demands placed on analog antialiasing filters. This strategy involves gathering a surplus of samples over a specific time, surpassing strict necessity. Consequently, the ADC acquires an augmented dataset, aiding in the mitigation of noise through averaging and bolstering the resolution of the eventual digital output.
Noise Shaping
Following the oversampling process, noise shaping comes into play. This phase orchestrates a distinct modulation of the quantization noise distribution. Unlike a uniform scattering of quantization noise across the frequency spectrum, noise shaping endeavors to shift this noise to higher frequencies. This alteration is facilitated by the Delta-Sigma modulator's intricate composition, encompassing the integrator, 1-bit DAC, and comparator. Through an ingenious feedback mechanism, the quantization error is looped back into the input via the integrator. This mechanism bestows an evolution upon the noise spectrum, effecting a reduction in noise power within the frequency band of interest while concurrently augmenting it at higher frequencies.
Decimation
Decimation, the conclusive stage in a Delta-Sigma ADC's operation, follows noise shaping and targets the elimination of excessive high-frequency quantization noise. Executed through a digital low-pass filter, decimation substantially reduces noise levels, leading to an improved signal-to-noise ratio (SNR) and overall performance. The extent of SNR improvement corresponds to 110log10(K), with K being oversampling ratio (K). Simultaneously, decimation addresses the surplus data rate resulting from prior oversampling by intelligently down-sampling to a more appropriate level through the selection of all nth samples, which not only aligns with operational needs but also heightens resolution within the pertinent frequency range.
Advantages of Delta-Sigma ADCs
Delta-Sigma ADCs have various advantages over other ADCs due to their specific architecture and operating principles. The most noteworthy benefits are excellent resolution and noise reduction, which make them especially well-suited for applications that need precision and accuracy.
High Resolution
One notable advantage of Delta-Sigma ADCs is their capacity to achieve high resolution. Resolution refers to the smallest distinguishable change in the output based on a change in the input, usually measured in bits. Delta-Sigma ADCs employ oversampling to gather more data points from the analog input signal. As previously mentioned, this involves sampling at a rate significantly higher than the Nyquist rate. Alongside noise shaping, which reorganizes quantization noise, this elevated sampling rate enables the effective number of bits (ENOB) to surpass what would be attainable through a conventional sampling process. As a result, Delta-Sigma ADCs are proficient at detecting very subtle alterations in the input signal. This attribute makes them exceptionally well-suited for applications such as audio processing and instrumentation, where high precision is required.
Noise Reduction
Noise mitigation stands as another prominent benefit offered by Delta-Sigma ADCs. In the process of converting analog signals to digital, quantization noise emerges due to the limited precision of the digital format. Delta-Sigma ADCs tackle this by leveraging the feedback loop of the modulator to naturally shape this noise, pushing it towards higher frequencies that lie beyond the desired frequency range. Subsequently, the decimation filter effectively eliminates these elevated-frequency noise components. This technique, known as noise shaping, constitutes a fundamental aspect of Delta-Sigma ADC operation. By curbing noise within the intended frequency range, Delta-Sigma ADCs markedly enhance the signal-to-noise ratio (SNR), thereby presenting a more refined and precise rendering of the original input signal.
Disadvantages of Delta-Sigma ADCs
While Delta-Sigma ADCs are highly recognized for their high resolution and noise reduction capabilities, there are several downsides to consider depending on the application. Speed limitations and complexity are two major drawbacks.
Speed Limitations
Delta-Sigma ADCs engage in a sophisticated sequence of operations, encompassing oversampling, noise shaping, and decimation. These operations are computationally demanding and inherently slower when contrasted with processes in alternative ADC types such as Successive Approximation Register (SAR) or Pipeline ADCs. To be specific, the elevated oversampling rate necessitates a surplus of samples beyond the minimal requirement, which in turn demands substantial processing. Consequently, this inherent intricacy introduces latency in the conversion procedure, rendering it unsuitable for swifter or real-time applications. Furthermore, the decimation process contributes added latency due to the involved filtering and down-sampling stages. Hence, for scenarios requiring rapid data acquisition, Delta-Sigma ADCs are not typically the favored selection.
Complexity
Delta-Sigma ADCs exhibit notably greater complexity in their architecture compared to other ADC variants. The incorporation of a feedback loop within the modulator, along with the essential inclusion of decimation filtering, imparts a heightened level of intricacy to the design and realization of these converters. This elevated complexity contributes to heightened manufacturing costs and can also pose hurdles during the phases of design and integration into broader systems. The requirement for meticulous calibration and tuning procedures to achieve optimal performance adds yet another layer of complexity. Furthermore, a comprehensive grasp of mathematical principles for optimizing noise shaping and filtering techniques demands a significant investment of time and effort.
Applications Suited for Delta-Sigma ADCs
Despite their drawbacks, Delta-Sigma ADCs are particularly well-suited for applications that can benefit from their high resolution and noise reduction capabilities. This section will look at two key situations where Delta-Sigma ADCs are especially useful: audio processing and precision measurement.
Audio Processing
Audio signals, whether collected by microphones or created by musical instruments, are essentially analog. A fundamental necessity in audio systems is the conversion of analog signals to digital forms for processing, storage, or transmission with minimal loss of fidelity. For various reasons, delta-sigma ADCs are a great choice for this application.
To begin with, the excellent resolution of Delta-Sigma ADCs is suitable for recording the subtle subtleties of audio signals. Because these ADCs oversample, they collect far more information per unit of time than is required, and noise shaping spreads quantization noise across a broad spectrum, allowing for a greater Signal-to-Noise Ratio (SNR) in the audio band.
Furthermore, noise shaping significantly reduces in-band noise, which is crucial in audio applications for preserving sound quality and integrity.
Finally, the common bandwidths of relevance in audio processing are rather modest (20 Hz to 20 kHz), implying that the speed limits of Delta-Sigma ADCs are insignificant in this context.
Precision Measurement
Precision measurement applications such as sensor data acquisition, instrumentation, and industrial process control require high accuracy and noise immunity. Delta-Sigma ADCs are especially well-suited for these tasks.
Delta-Sigma ADCs' excellent resolution ensures that extremely minor changes in the input signal can be accurately caught. This is critical in precision measurement applications where even little signal variations can be significant.
In addition, Delta-Sigma ADCs use a noise-shaping technique that keeps quantization noise out of the frequency band of relevance. This is especially significant in measurement systems with low-level signals because high noise levels might hide the signal of interest. Because many precision measurement applications (such as temperature logging or strain gauge measurements) are quite slow, the speed limits of Delta-Sigma ADCs have little negative impact on performance.
Design Considerations and Trade-offs
Several essential considerations and trade-offs must be taken into account when developing a system that uses Delta-Sigma ADCs, this is to guarantee that the ADC matches well with the application's needs. These factors include resolution, bandwidth, complexity, power consumption, and cost. In this section, we will go through each of these points in depth.
Resolution and Oversampling Ratio: The oversampling ratio (OSR) determines the resolution of a Delta-Sigma ADC. Higher OSR yields higher resolution at the tradeoff of decreased bandwidth and increased computation. As a result, it is critical to determine an ideal OSR that meets the resolution requirement without needlessly lowering bandwidth or raising the processing overhead.
Bandwidth: A Delta-Sigma ADC's bandwidth is inversely proportional to its OSR. High-resolution applications may need a high OSR, which limits bandwidth naturally. In applications that require both high resolution and vast bandwidth, this trade-off might become a substantial constraint. As a result, it is critical to properly balance the OSR and ADC bandwidth based on the application's individual requirements.
Complexity and Filter Design: Due to noise shaping and decimation filters, Delta-Sigma ADCs have a more sophisticated architecture than other ADC types. The complexity of the filter design, particularly the decimation filter, can have a substantial impact on the performance of the ADC and as such must be taken into account. The complexity of Delta-Sigma ADCs may be a disadvantage in applications where simplicity and low latency are crucial.
Power Consumption: Oversampling and noise shaping need more computations, which might result in greater power consumption. The power consumption of the Delta-Sigma ADC must be examined and optimized for the individual application in battery-powered applications or circumstances where power efficiency is crucial.
Cost: Delta-Sigma ADCs are frequently more expensive than simpler ADC architectures due to their complexity. However, increased costs may be justified in applications requiring high resolution and low noise. It is critical to examine the budget limits and balance them against the performance needs of the application.
Implementation Choices: Depending on the application, designers may choose discrete Delta-Sigma ADC components or integrated solutions that combine the ADC with other functions (such as microcontrollers). The decision between these solutions will be influenced by factors such as available space, economic concerns, and the need for integration with other system components.
直接登录
创建新帐号