Introduction to ADCs in Communication Systems
Role and Importance
Communication systems lie at the core of the contemporary information era, facilitating the exchange of data using various channels such as radio waves, wired cables, and optical fibers. Analog-to-digital converters (ADCs) assume an essential function within modern communication systems. Whether it's within the realm of cellular phones, Wi-Fi routers, Ethernet networks, or optical communication setups, ADCs hold a pivotal role in signal processing.
Within a typical communication system, information takes the form of an analog signal as it traverses a medium. This analog signal can represent a range of data types, encompassing voice, data, images, or any other informational content. Prior to its digital processing, storage, or transmission, this information must undergo a transformation into a digital format. This critical conversion process is where ADCs come into play, converting the analog signals into discrete digital bits. While the subsequent step involves the conversion of these digital bits back into an analog signal on the receiving end, the primary focus remains on the indispensable role of ADCs in the initial conversion stage.
Typical ADC Requirements for Communication Systems
Resolution: Within communication systems, the resolution of an ADC stands as a pivotal specification. It signifies the degree of precision with which the ADC can discretize an incoming analog signal. A heightened resolution becomes imperative in scenarios where the signal exhibits a broad dynamic range or when even slight alterations in the signal necessitate precise representation.
Sample Rate: The sampling rate serves as another critical parameter. It defines the frequency at which the ADC samples the input analog signal. Adhering to the Nyquist theorem, for accurate signal representation, the sampling rate must be at least twice the highest frequency component of the input signal. In the realm of communication systems, especially within high-frequency applications such as radio and optical communications, elevated sampling rates become indispensable.
Linearity: In communication systems, it is imperative for the ADC to uphold a linear correlation between the input analog signal and the resultant digital representation. Deviations from linearity can introduce distortion, thereby impacting the quality of the transmitted information.
Power Consumption: Efficiency is often a paramount concern in communication systems, particularly in the context of portable and battery-powered devices. Hence, careful consideration of the ADC's power consumption is crucial.
Noise Performance: The ADC should exhibit minimal noise characteristics as noise can profoundly affect communication integrity. The Signal-to-Noise Ratio (SNR) stands as a common metric employed to assess noise performance.
Bandwidth: The ADC must possess ample bandwidth to accommodate the frequency range pertinent to the communication system's objectives.
Comprehending the specific requisites of the given communication system becomes pivotal in the process of selecting or designing an ADC with the requisite attributes. The ADC constitutes a fundamental cornerstone within communication systems, necessitating optimization concerning performance, power efficiency, and cost alignment with the application's demands.
ADCs in Wireless Communications
Role in Software-Defined Radios
Software-defined radios (SDRs) have ushered in a transformative era in the construction and deployment of radio communication systems. Diverging from conventional hardware-based radio systems, SDRs leverage software to execute signal-processing tasks that were traditionally within the domain of hardware. This adaptability empowers a single SDR platform to accommodate multiple communication standards and to undergo reconfiguration or enhancement via software updates.
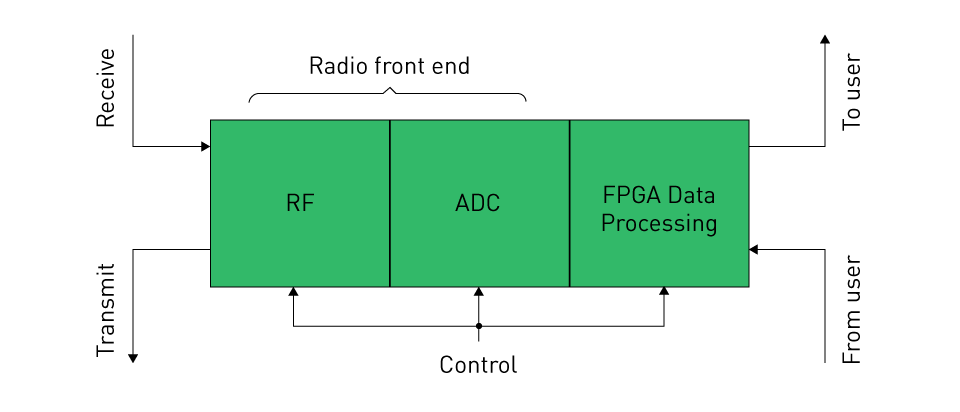
Figure 4: ADC in SDR transceiver
In the realm of SDRs, ADCs assume a pivotal role as critical components. In the typical SDR setup, the antenna intercepts an analog signal, which can encompass various frequencies and modulation schemes. The analog front-end is responsible for filtering and amplifying this signal, after which it undergoes conversion into a digital format courtesy of an ADC. Following this digitization, software-based digital signal processing algorithms come into play, facilitating the extraction of specific information, such as signal demodulation or the elimination of unwanted interference.
In essence, within an SDR, the ADC serves as the vital link between the analog RF domain and the digital processing sphere. The presence of ADCs is paramount, as it underpins the flexibility and adaptability integral to the functionality of SDRs. Without ADCs, the remarkable versatility and reconfigurability inherent to SDRs would remain unattainable.
ADC Specifications for Cellular and Wi-Fi Applications
In the realm of cellular and Wi-Fi applications, the demands placed on ADCs are notably rigorous, given the imperative for high data rates, expansive bandwidths, and the assurance of dependable communication in environments characterized by substantial noise. Here, we delineate some pivotal ADC specifications pertinent to these applications:
Sampling Rate: In the context of high data rate communication standards such as 4G, 5G, and Wi-Fi, the ADC must possess the capability to sample at exceedingly high rates. For instance, in order to accommodate the 100 MHz bandwidth associated with certain 5G signals, the ADC necessitates a minimum sampling rate of 200 Msps (Mega Samples Per Second).
Resolution: The resolution of the ADC stands as a decisive factor in shaping the signal-to-quantization noise ratio. A heightened resolution, signifying a greater number of bits, augments the faithfulness of the digital representation of the analog signal. In the arena of cellular and Wi-Fi applications, it is customary to encounter resolutions ranging from 12 to 16 bits as the norm.
Dynamic Range: The ADC's ability to accommodate a wide spectrum of signal levels without introducing distortion emerges as a vital requirement. This assumes heightened significance, especially within cellular systems where the ADC must contend with exceedingly weak signals emanating from distant cell towers, alongside robust signals originating from nearby sources.
Linearity: The attainment of superior linearity becomes imperative to safeguard against signal distortion attributable to the ADC. This holds particular prominence within communication systems, wherein non-linearities could precipitate interference between channels.
Power Consumption: In the domain of mobile devices like smartphones, power consumption occupies a paramount position. The ADC should adeptly execute its requisite functions while minimizing power consumption to maximize the longevity of battery life.
Noise Performance: Within the realm of wireless communications, the environment often teems with noise. Accordingly, the ADC must exhibit commendable noise performance characterized by low jitter and a high Signal-to-Noise Ratio (SNR) to faithfully digitize signals in the face of challenging noise conditions.
ADCs in Wired Communication Systems
Applications in High-Speed Data Transmission
Wired communication systems continue to serve as indispensable infrastructure across a spectrum of industries, data centers, and residential settings. As the demand for higher data transmission rates escalates, there is a concomitant surge in the requirement for ADCs capable of managing the rigors of high-speed data transmission within wired communication systems.
One salient arena where ADCs play a pivotal role in wired communication is within Ethernet networks. The domain of high-speed Ethernet, particularly within data centers and backbone networks mandates the deployment of high-performance ADCs to support gigabit and10-gigabit per second data rates. In this context, ADCs assume the task of converting analog signals received via twisted-pair cables or optical fibers into digital data streams amenable to processing by network equipment.
Another notable application resides within digital subscriber line (DSL) modems, where ADCs assume an indispensable role in the conversion of analog signals stemming from telephone lines into digital data, facilitating the provision of broadband internet services.
Moreover, Universal Serial Bus (USB) interfaces, ubiquitous for linking peripherals to computers, also harness the capabilities of ADCs. For example, within audio interfaces and external sound cards, ADCs execute the conversion of analog audio signals into digital data, which is then transmitted across the USB connection.
ADC Requirements for Ethernet, USB, and other standards
ADCs must meet specific performance requirements for high-speed wired communication systems like Ethernet and USB interfaces to guarantee dependable data transmission:
High Sampling Rates: ADCs must be capable of sampling at extremely high frequencies in order to support high data rates. This could require sampling rates in the range of Giga samples per second (Gsps) for 10 Gigabit Ethernet.
Resolution: For wired communications, a modest resolution is typically sufficient; nonetheless, the resolution still affects the quantization noise and, eventually, the bit error rate. Resolutions typically range from 8 to 14 bits.
Jitter Performance: Timing precision is essential for high-speed data transfer. To reliably sample the incoming signals at precise intervals, ADCs must have low jitter.
Power Efficiency: Power consumption can be a serious problem in sizable data centers. ADCs used in such contexts should therefore be power-efficient in order to lower the system's overall power consumption.
Linearity and Distortion: High linearity guarantees that the ADC causes less distortion to the signal, which is essential for ensuring signal integrity in high-speed communication systems.
Integration and Footprint: In order for ADCs to fit into small devices, particularly in consumer applications like USB interfaces, they must be highly integrated and have a small footprint.
ADCs in Optical Communication Systems
Role in Fiber Optic Transceivers
Optical communication systems have ushered in a revolution in data transmission, offering the capability for exceptionally high data rates over extensive distances. These systems predominantly rely on optical fibers as the transmission medium. Within these optical communication setups, fiber optic transceivers emerge as pivotal components, serving as the vital interface between the optical network and the electrical network. They facilitate the conversion of optical signals into electrical signals and vice versa. ADCs assume a central role within this conversion process, particularly in the reception path where optical signals undergo transformation into electrical signals.
In the reception pathway, the journey commences with the conversion of the optical signal into an electrical counterpart through the agency of a photodetector. This electrical signal, though analog in nature, necessitates transformation into a digital format to facilitate processing or transmission across a digital network. Herein, the ADC assumes a position of paramount importance, facilitating the conversion of the analog electrical signal into a digital representation. This conversion process must exhibit a high degree of precision to safeguard the integrity of the transmitted data, underscoring the criticality of the ADC's role in optical communication systems.
Considerations for High-Speed, High-Resolution ADCs
When choosing and deploying ADCs for fiber optic transceivers, there are numerous crucial factors to take into account:
High Sampling Rates: Optical communication systems frequently work at data rates of 10 Gbps to 100 Gbps or more, or more. ADCs with Giga samples per second (Gsps) sampling rates are necessary to properly sample the incoming high-speed signals.
Resolution: A higher-resolution ADC is instrumental in representing the input signal with greater precision and fidelity. This heightened accuracy becomes of paramount importance, especially in the context of high-speed data transmission as it plays a crucial role in preserving the integrity of the transmitted data. ADCs with resolutions of 8 bits or greater are typical for optical communication systems.
Jitter and Noise Performance: As data rates reach high levels, the precision of timing becomes a critical factor. ADCs employed in optical communication systems must exhibit low jitter to accurately sample signals at these elevated speeds. Additionally, maintaining low noise levels is imperative to ensure the signal-to-noise ratio (SNR) remains high, particularly when transmitting over extended distances.
Bandwidth: The ADC's input bandwidth must align with, or even exceed the signal bandwidth in optical communications. This implies that ADCs with several Gigahertz of bandwidth may be requisite for these applications to effectively handle the wide spectrum of optical signals.
Power Consumption and Heat Dissipation: In expansive optical networks like data centers, power consumption and heat generation can pose substantial challenges. Consequently, opting for power-efficient ADCs becomes a crucial consideration in such scenarios to manage energy usage and mitigate heat-related issues.
Integration with Other Components: Within optical communication systems, seamless integration with complementary components such as amplifiers, clock sources, and digital signal processors assumes paramount importance. ADCs that can be readily incorporated into transceiver modules or other system components are highly preferred to streamline system design and deployment.
直接登录
创建新帐号