Advancements in AC Power Generation
Cutting-edge Research in Improving Efficiency and Sustainability in AC Power Generation
As a result of the pressing requirement to improve both efficiency and sustainability, the sector of alternating current (AC) power generation is currently undergoing a considerable transition. In order to minimize energy waste and negative environmental effects, cutting-edge research focuses on improving the efficiency of power generation processes. Important fields of inquiry include the following:
Advanced Turbine Technologies: Research is being done to increase the efficiency of gas and steam turbines, which are essential components of many power plants. The purpose of this research is to develop advanced turbine technologies. It is possible for advancements in turbine blade materials and aerodynamic designs to result in increased thermal efficiency and decreased fuel consumption due to these innovations.
Combined Heat and Power (CHP) Systems: CHP systems, which are often referred to as cogeneration, are able to generate both electricity and heat that may be put to beneficial use from the same original source of energy. In contrast to conventional power plants, which waste a considerable proportion of energy as heat, combined heat and power (CHP) systems are able to capture this heat and put it to profitable use by, for example, heating buildings, providing hot water, or powering industrial processes. Through the development of more efficient combustion processes and the incorporation of renewable energy sources, the research endeavors to improve the efficiency of combined heat and power (CHP) systems.
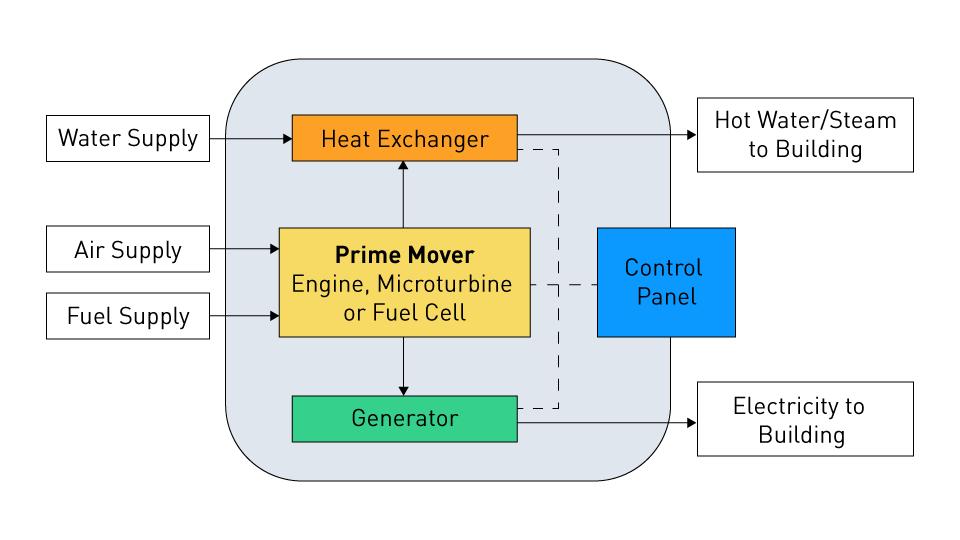
Figure 1: A packaged CHP system
Carbon Capture and Storage (CCS): Technologies that capture and store carbon are currently being developed in order to reduce the negative effects that fossil fuel-based electricity generation has on the environment. These technologies are able to collect carbon dioxide emissions from power stations and store them underground, keeping them from entering the atmosphere.
Artificial Intelligence (AI) and Machine Learning (ML): AI and ML are being utilized to improve the efficiency of power plant operations. Through the analysis of huge amounts of operational data, these technologies are able to perform tasks such as predicting the breakdown of equipment, optimizing maintenance schedules, and improving overall plant efficiency.
Developments in Renewable Energy Technologies and Their Integration into AC Power Systems
In the current study, one of the primary focuses is on the incorporation of renewable energy sources into alternating current power systems. The objective of this research is to address difficulties that are associated with variability, reliability, and efficiency. Important advancements include the following:
Photovoltaic (PV) Solar Power: Research in photovoltaic (PV) solar power aims to improve the efficiency of solar panels and lower the manufacturing expenses. The development of new materials, including perovskite and multi-junction solar cells, holds the promise of improved performance and increased conversion efficiencies under a wide range of environmental circumstances. In addition, developments in solar tracking systems have made it possible to capture more solar energy with greater efficiency throughout the day.
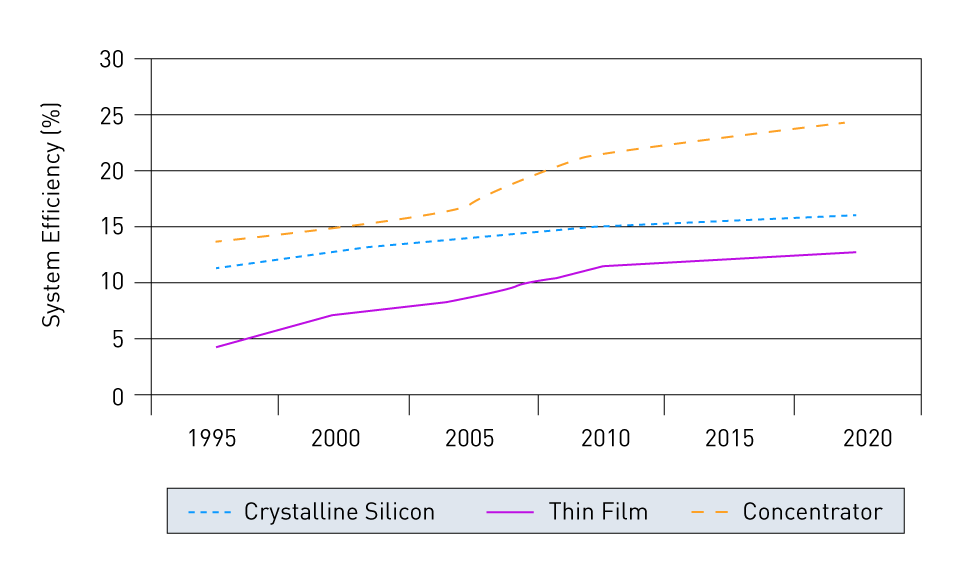
Figure 2: PV efficiency improvement with time
Wind Power: The research that is being done in the field of wind energy strives to improve the effectiveness and dependability of wind turbines. A number of advancements have been made, including increased rotor diameters, more sophisticated blade designs, and enhanced turbine control systems. Researchers are also exploring offshore wind farms to harness the more powerful and reliable wind resources available at sea.
Hydropower: The main focus of improvements in hydropower technology is to reduce negative environmental effects and increase operational effectiveness. Innovations include the deployment of small-scale, modular hydropower systems in diverse geographical regions, and the creation of fish-friendly turbine designs.
Energy Storage Systems: Researchers are conducting extensive research on energy-storing devices to address the intermittent nature of renewable energy sources. For grid-scale applications, battery storage technologies, and lithium-ion batteries in particular, are now undergoing performance optimization. Supercapacitors, flywheels, and pumped hydroelectric storage are some of the other many storage systems that are currently being investigated by researchers.
Grid Integration and Smart Grids: The incorporation of renewable energy sources into preexisting alternating current (AC) power systems necessitates the utilization of advanced grid management strategies. Smart grid technologies, which include enhanced metering infrastructure, demand response, and distributed energy resource management systems, make it possible for grid operations to be more efficient and flexible. The development of grid synchronization techniques and the enhancement of grid stability are also currently the primary focuses of research in order to support large levels of renewable energy penetration.
Hybrid Power Systems: Hybrid power systems integrate elements from a number of different renewable energy sources, including solar, wind, and hydropower, in order to produce a power supply that is more consistent and dependable. The goal of the research being conducted is to build integrated control systems that are capable of managing the fluctuation of renewable energy generation and to optimize the interaction between various sources.
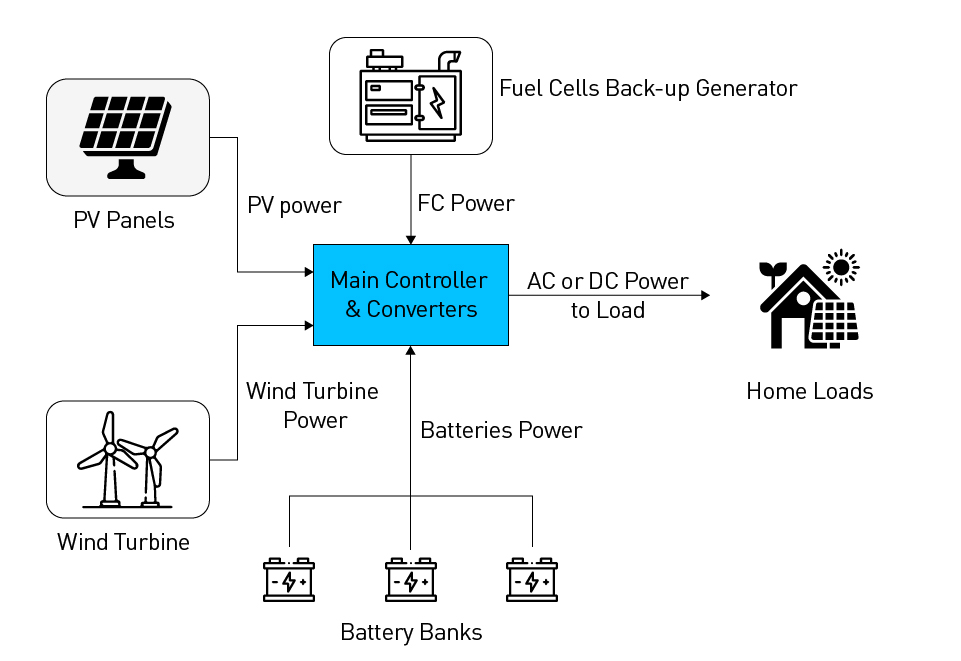
Figure 3: Hybrid power system concept
Microgrids: Microgrids are localized power networks that are capable of functioning independently or in cooperation with the main grid. Microgrids are also known as microgrids. Research is currently underway on the design, control, and optimization of microgrids to enhance their capacity to incorporate renewable energy sources and provide reliable power in underserved or remote locations.
Innovations in Transmission and Distribution
Developments in High Voltage Direct Current (HVDC) and Flexible AC Transmission Systems (FACTS) are significantly altering the landscape of power transmission. Traditional AC transmission systems have limits that can be addressed by these technologies, which offer improved efficiency, reliability, and flexibility in power transmission.
High Voltage Direct Current (HVDC)
Electricity transmission over long distances and with high capacities widely utilizes high-voltage direct current (HVDC) technology. The use of direct current in high-voltage direct current (HVDC) systems, as opposed to alternating current (AC) transmission, results in fewer losses over longer distances. The following are some of the primary benefits of HVDC:
Reduced Transmission Losses: When compared to AC systems, HVDC systems have fewer losses related to transmission. This can be attributed to a variety of factors, including the absence of any skin effect and the reduction of reactive power losses. Due to this advantage, high-voltage direct current (HVDC) systems are ideally suited for the transmission of power over extensive distances, such as from rural renewable energy sources to center cities.
Enhanced Stability and Control: High-voltage direct current (HVDC) enables precise control of power flow, which in turn improves grid stability and reduces the likelihood of blackouts. The overall reliability of the grid is improved when there is the capability to make rapid adjustments to the flow of power.
Cost-Effectiveness: A high-voltage direct current (HVDC) power circuit typically requires only two conductors, whereas an alternating current (AC) power circuit can require three. In light of this, high-voltage direct current (HVDC) becomes more cost-effective over long distances as a result of decreased energy losses and cheaper infrastructure needs (for example, fewer and thinner cables).
Interconnection of Different Grids: High-voltage direct current (HVDC) makes it possible to connect asynchronous alternating current (AC) grids, which in turn makes it possible to trade power between regions that have different frequencies or standards. When it comes to developing electricity networks that are more resilient and linked, this skill is absolutely necessary.
Flexible AC Transmission Systems (FACTS)
In order to improve the controllability and capacity of AC transmission networks, a family of technologies known as FACTS has been developed. These technologies are based on power electronic devices. Through the dynamic regulation of power flows and voltage levels, FACTS devices contribute to an increase in the efficiency and stability of the power grid infrastructure. Some of the most notable FACTS technologies are:
Static VAR Compensators (SVC): SVCs are responsible for providing dynamic reactive power compensation, which helps to improve power factor, maintain voltage stability, and enhance power quality. It is also helpful in minimizing voltage variations brought on by fluctuating renewable energy sources for which they are particularly valuable.
Static Synchronous Compensators (STATCOM): They are a type of voltage control device that is used to offer reactive power compensation in a quick and effective manner. By providing support for voltage levels during transient disturbances and load shifts, they contribute to the enhancement of grid stability.
Unified Power Flow Controllers (UPFC): These are devices that simultaneously regulate voltage, impedance, and phase angle in order to provide comprehensive control over the flow of power in transmission lines. By allowing for greater flexibility, current transmission infrastructure may be utilized more effectively, which in turn reduces congestion concerns.
Table 1: FACTS device comparison
Feature | Static VAR Compensators (SVC) | Static Synchronous Compensators (STATCOM) | Unified Power Flow Controllers (UPFC) |
---|---|---|---|
Primary Function | Voltage control and stability | Voltage control and stability | Voltage control, power flow control |
Operating Principle | Utilizes thyristor-controlled reactors and capacitors | Makes use of voltage-source converters | A combination of series and shunt compensation |
Reactive Power Range | Fixed by design | Flexible, wide range | Highly flexible |
Response Time | Slower (due to thyristor switching) | Fast (due to IGBT/voltage-source converter) | Fast |
Control Flexibility | Less flexible, more discrete steps | Highly flexible, continuous control | Highly flexible |
Harmonics | Produces harmonics, needs filters | Lower harmonic generation | Lower harmonic production |
Installation Complexity | Less complex and established | More complex than SVC | Most complex |
Cost | Generally lower | Higher than SVC | Highest |
Space Requirement | Larger footprint | Smaller footprint | Larger footprint (due to combination of devices) |
Applications | Voltage stabilization, flicker control, reactive power compensation | Voltage stabilization, dynamic response, reactive power compensation | Comprehensive power flow control, voltage stabilization, and dynamic response |
Dynamic Performance | Good for steady-state control | Suitable for dynamic and transient control | Excellent |
Power Quality Improvement | Moderate | High | High |
System Impact | Confined to reactive power control | Enhanced reactive power control | Enhanced control of active and reactive power |
Complexity of Control Systems | Less complex | More complex | Most complex |
Typical Use Cases | Industrial plants, transmission lines | Wind farms, solar plants, transmission lines | Major grid interconnections, complex power flow scenarios |
Smart Distribution Network Technologies and their Role in Enhancing Grid Flexibility
The evolution of smart distribution networks is becoming increasingly important in the process of changing conventional electricity grids into systems that are more resilient, efficient, and flexible. By utilizing cutting-edge technologies, smart distribution networks are able to enhance the management and operation of electricity distribution. This, in turn, makes it easier to include distributed energy resources (DERs) and improves grid performance.
Among the most important aspects and advantages of smart distribution networks are:
Advanced Metering Infrastructure (AMI): AMI refers to the implementation of smart meters, which present information in real time regarding the amount of electricity that is consumed. With this information, utilities are able to adopt dynamic pricing, detect power shortages in a timely manner, and improve their tactics for responding to demand.
Distributed Energy Resource Management Systems (DERMS): Solar panels installed on rooftops, battery storage systems, and electric vehicles are all examples of distributed energy resources that can be managed by Distributed Energy Resource Management Systems (DERMS). These systems coordinate and optimize the operation of these distributed energy resources. DERMS works to improve grid stability and enable more efficient use of renewable energy sources by successfully integrating distributed energy resources (DERs).
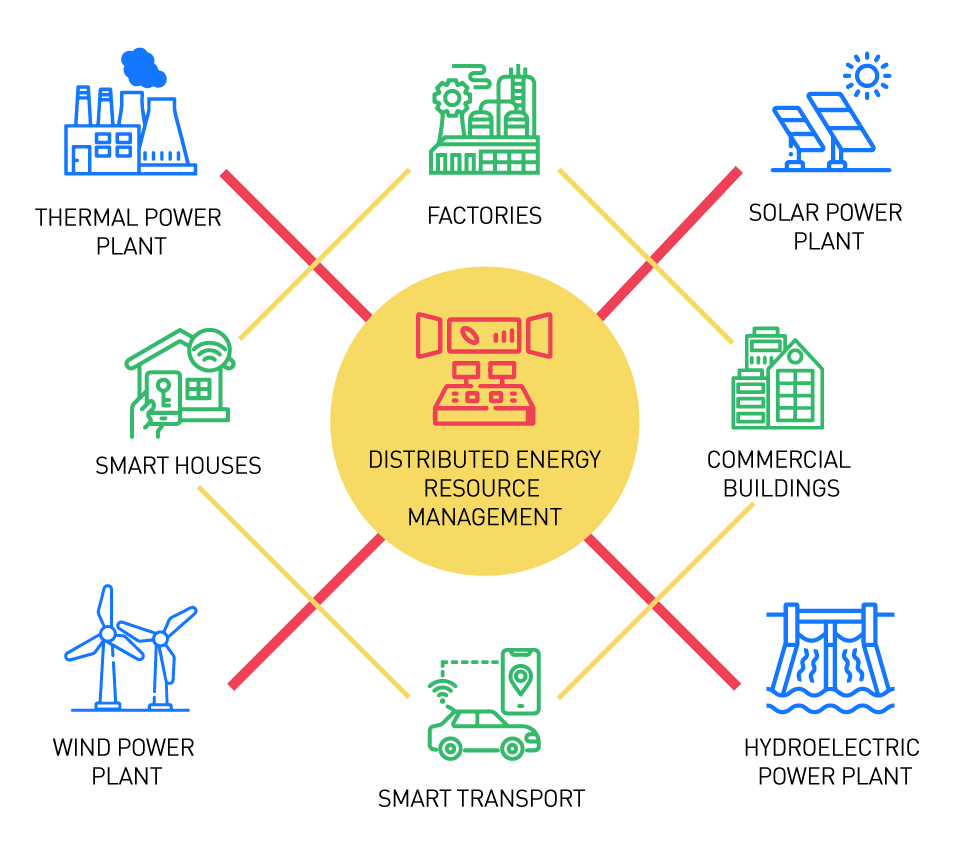
Figure 4: DERMS concept
Automated Distribution Management Systems (ADMS): These systems provide extensive control over distribution networks by means of real-time monitoring, fault detection, and automated switching. Reliability is improved by these systems because they promptly isolate faults and restore power, hence minimizing the length of time that power outages last and the impact they have.
Grid Edge Technologies: Innovations at the grid edge, including sophisticated sensors, communication networks, and edge computing, make it possible to regulate and monitor distribution networks with greater precision. These technological advancements contribute to the decentralized nature of power generation and improve the flexibility of the grid.
Demand Response and Load Management: Smart distribution networks enable demand response programs, which incentivize users to curtail or adjust their electricity usage during peak periods, while also supporting load management procedures. This capacity helps maintain a balance between supply and demand, which in turn reduces the requirement for costly peaking power plants and improves the overall efficiency of the entire system.
Role in Enhancing Grid Flexibility: Distribution networks that are intelligent play a crucial part in meeting the ever-increasing penetration of renewable energy sources and the ever-changing demands of modern consumers of electricity. The integration of variable renewable energy is improved, operational costs are reduced, and the overall stability and resilience of the grid are increased as a result of these networks. This is accomplished by increasing the visibility and control of distribution systems.
Emerging Materials and Technologies
The discovery of new materials is at the forefront of technological breakthroughs in alternating current (AC) power systems. These advancements have the potential to transform the ways in which electricity is generated, transmitted, and utilized. There are two major kinds of new materials that have a great deal of promise: nanomaterials and superconductors.
Superconductors
After being cooled to extremely low temperatures, materials are referred to as superconductors when they are able to conduct electricity with zero resistance. Because of this feature, power transmission can be carried out in an extremely efficient manner, and energy losses can be greatly reduced. In AC power systems, the following are some examples of applications of superconductors:
High-Temperature Superconductors (HTS): In comparison to conventional superconductors, high-temperature superconductors (HTS) are characterized by their ability to function at temperatures that are significantly higher. HTS materials have recently achieved considerable advancements. The materials in question enable the creation of superconducting cables and fault current limiters that can carry larger current densities and provide strong grid protection.
Superconducting Magnetic Energy Storage (SMES): SMES devices are able to store energy in the magnetic field that is produced when direct current is passed through a superconducting coil. The quick discharge of energy that these systems are capable of delivering enables them to provide immediate power support during times of peak demand or grid interruptions.
Superconducting Generators and Transformers: The utilization of superconducting materials in generators and transformers has the potential to result in devices that are more compact, lightweight, and effectively efficient. Through their ability to manage higher power densities, these components contribute to an overall improvement in the efficiency and dependability of power systems.
Nano-Materials
Due to their unique properties at the nanoscale, nanomaterials have the potential to enhance the effectiveness and efficiency of alternating current power systems. Among the most important applications are:
Nanocomposites for Insulation: Nanocomposite materials, which blend nanoparticles with standard insulating materials, offer higher dielectric characteristics and thermal stability. Nanocomposites are used for insulation. These materials improve the insulating performance of cables, transformers, and other components of power systems, which ultimately results in greater dependability and endurance.
Carbon Nanotubes (CNTs): In addition to their remarkable electrical conductivity, mechanical strength, and thermal conductivity, carbon nanotubes (CNTs) are also known for their exceptional mechanical strength. Conductors that are lightweight and have a high capacity can be developed with their help, and the performance of power transmission lines and electrical interconnects can be improved with their availability.
Graphene-Based Materials: Graphene, which is a single sheet of carbon atoms organized in a hexagonal lattice, possesses exceptional electrical, thermal, and mechanical properties. Applications for graphene-based materials include energy storage devices, conductive coatings, and enhanced sensors for power system monitoring.
Technological Breakthroughs in Power Electronics and their Applications in AC Power Systems
Power electronics are experiencing major technological developments, which are generating considerable improvements in the efficiency, dependability, and control of alternating current (AC) power systems. These innovations are making it possible to integrate renewable energy sources in a more efficient manner, improving grid stability, and streamlining the processes involved in power conversion.
Wide Bandgap Semiconductors: Wide bandgap (WBG) semiconductors, which include silicon carbide (SiC) and gallium nitride (GaN), provide improved performance in comparison to conventional silicon-based devices. The operation of WBG semiconductors at higher voltages, frequencies, and temperatures results in a number of advantages, including the following:
- Increased Efficiency: As a result of the lower switching losses and higher thermal conductivity that WBG devices exhibit, they are able to achieve increased efficiency in power conversion and minimize the amount of cooling that is required. Solar inverters, electric vehicle chargers, and industrial drives are all examples of applications that can benefit greatly from this efficiency.
- Compact Designs: Compact designs are made possible by the higher performance of WBG semiconductors, which enables the construction of power electronic devices that are either smaller or lighter in size. Portable power systems and electric vehicles are two examples of applications that benefit from this compactness because of the space constraints they present.
- Improved Reliability: The use of WBG materials results in increased durability and robustness, which in turn improves the reliability and longevity of power electronic components. Having this level of dependability is essential for applications that are of critical importance, such as grid infrastructure and renewable energy systems.
Advanced Converter and Inverter Technologies: The incorporation and exploitation of renewable energy sources can be optimized through the implementation of converter and inverter designs that have undergone significant and innovative changes. Most important developments include:
- Multi-Level Converters: When compared to typical two-level converters, multi-level converters provide superior power quality and a reduction in electromagnetic interference (EMI). Multi-level converters excel in high-voltage applications like high-voltage direct current (HVDC) transmission and large-scale solar farms.
- Bidirectional Inverters: Inverters that operate in both directions are known as bidirectional inverters. These inverters allow for the smooth transfer of electricity between the grid and energy storage devices. Applications such as grid-connected solar systems, battery energy storage, and vehicle-to-grid (V2G) technologies require this functionality.
- Smart Inverters: Smart inverters are characterized by their incorporation of sophisticated control algorithms and communication capabilities, which allow for the monitoring and management of power flows in real time. These inverters enable the integration of distributed energy resources (DERs), enhance grid stability, and support demand response programs.
Energy Harvesting and Wireless Power Transfer: Recent technological advancements in energy harvesting and wireless power transfer are introducing novel opportunities for the powering of remote sensors, Internet of Things devices, and electric cars.
- Energy Harvesting: The process of energy harvesting involves the utilization of technology that is capable of capturing ambient energy from many sources, including vibrations, thermal gradients, and electromagnetic waves. Power systems can benefit greatly from the utilization of these technologies for the purpose of powering wireless sensors and monitoring devices.
- Wireless Power Transfer (WPT): Wireless power transfer (WPT) refers to the collection of technologies that enable the transfer of power without the need for physical connections. These technologies include inductive and capacitive coupling. WPT is now being investigated for use in a variety of applications, including the charging of electric vehicles, the supply of contactless power in industrial environments, and the powering of medical implants.
直接登录
创建新帐号