Passive Power Factor Correction
Power factor adjustment, a crucial strategy in alternating current (AC) power systems, aims to boost the effectiveness and capacity of electrical networks. The passive power factor correction (PFC) method stands out among the different approaches to power factor correction due to the fact that it is one of the most straightforward, dependable, and cost-effective methods. Providing an understanding of the role that passive power factor correction plays in improving power system performance, this section looks into the uses of passive power factor correction as well as its limitations.
Passive PFC Circuits
Power factor correction using passive means makes use of passive components like inductors and capacitors in order to achieve the desired improvement in power factor. Arranging these components in this manner produces a low-pass filter, aiding in reducing the harmonic distortion present in the input current.
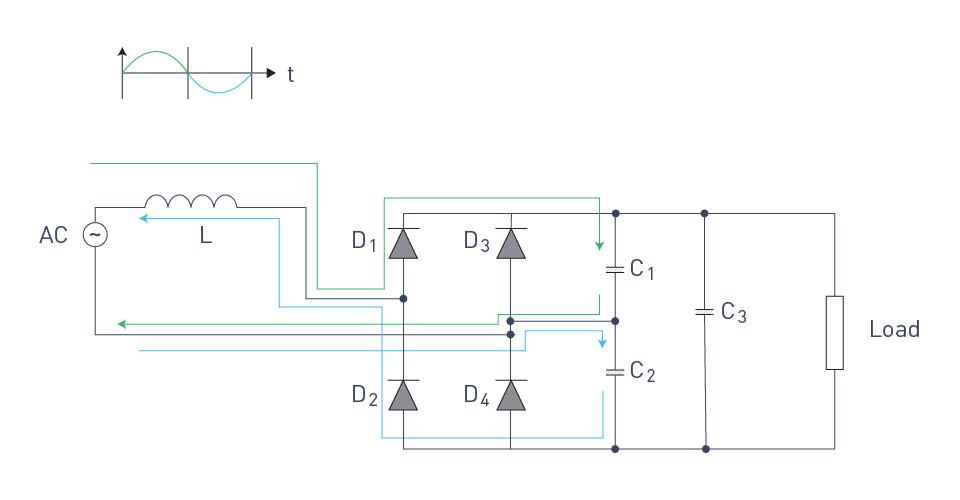
Figure 2: Passive PFC circuit with current paths
Simpleness, cost-effectiveness, and dependability are aspects that contribute to the advantages. However, their power factor correction is less effective, especially in situations with variable load conditions, and their bulkiness stems from the use of large-sized inductors and capacitors.
Applications of Passive Power Factor Correction
Industrial Motors and Machinery: In the context of industrial settings, where massive motors and machinery frequently operate with inductive loads that result in a low power factor, one of the principal uses of passive power factor correction (PFC) is found. Passive power factor correction (PFC) is a method that improves power factor by compensating for the reactive power demand. This is accomplished by integrating capacitors or inductor-capacitor (LC) circuits in parallel with these loads.
Lighting Systems: Passive photovoltaic cells are also utilized in lighting systems, namely in lighting systems that make use of inductive ballasts, such as fluorescent lighting. The addition of capacitors to the lighting circuit has the potential to decrease the amount of reactive power that is consumed, hence improving the overall efficiency of the lighting system.
HVAC Systems: Those Heating, Ventilation, and Air Conditioning (HVAC) systems that make use of a considerable number of inductive components are able to reap the benefits of passive power factor correction (PFC). It is possible to enhance the electrical efficiency of HVAC units by adjusting the power factor, which in turn reduces the amount of energy consumed and the costs associated with operations.
Distribution Networks: In order to enhance the power factor of the network, utilities and power distributors may choose to deploy passive power factor correction (PFC) at substations or along distribution lines. Through the utilization of this application, voltage levels may be managed, losses can be minimized, and the capacity of the electrical grid can be maximized.
Limitations of Passive Power Factor Correction
In spite of the fact that passive PFC has a great deal of benefits, it is not without certain restrictions that must be taken into consideration:
Fixed Compensation: Passive power factor correction (PFC) provides a fixed amount of reactive power compensation, which may not fully correlate with the load's fluctuating reactive power demand and requires some adjustment. This disparity can also result in under- or over-compensation, which is especially problematic for systems that experience changing loads.
Resonance Risk: The incorporation of capacitors into the power system has the potential to generate resonance situations at specific frequencies, which may result in overvoltages and damage to the equipment. To avoid resonance problems, careful design and analysis are required.
Maintenance and Aging: The performance of passive PFC components, particularly capacitors, can deteriorate over time due to aging and environmental variables. When this occurs, maintenance is necessary. It is vital to perform routine maintenance and replace components in order to guarantee that the PFC system will continue to function effectively over time.
Lack of Dynamic Control: On the other hand, passive power factor correction (PFC) systems, in contrast to active PFC approaches, do not possess the capability to dynamically react to changes in the power system that occur in real time. This constraint makes it less suitable for systems with loads that are extremely variable or that require precise control of the power factor.
Active Power Factor Correction
Active power factor correction, also known as PFC, is a complex method for optimizing the power factor in alternating current (AC) power systems. It makes use of electronic management technologies to dynamically change and improve power quality. Active power factor correction contrasts with passive power factor correction, which is based on static capacitors or inductor-capacitor (LC) circuits. Active PFC makes use of power electronics to accurately manage the amount of compensatory reactive power in real time. This section delves into the applications and outcomes of active power factor correction, specifically highlighting its role in modern electrical networks.
Overview of Different Active PFC Topologies
Active PFC employs electronic components like integrated circuits and switching devices such as MOSFETs. These components actively adjust the input current, enabling it to align more accurately with the input voltage waveform, thus achieving a near-unity power factor.
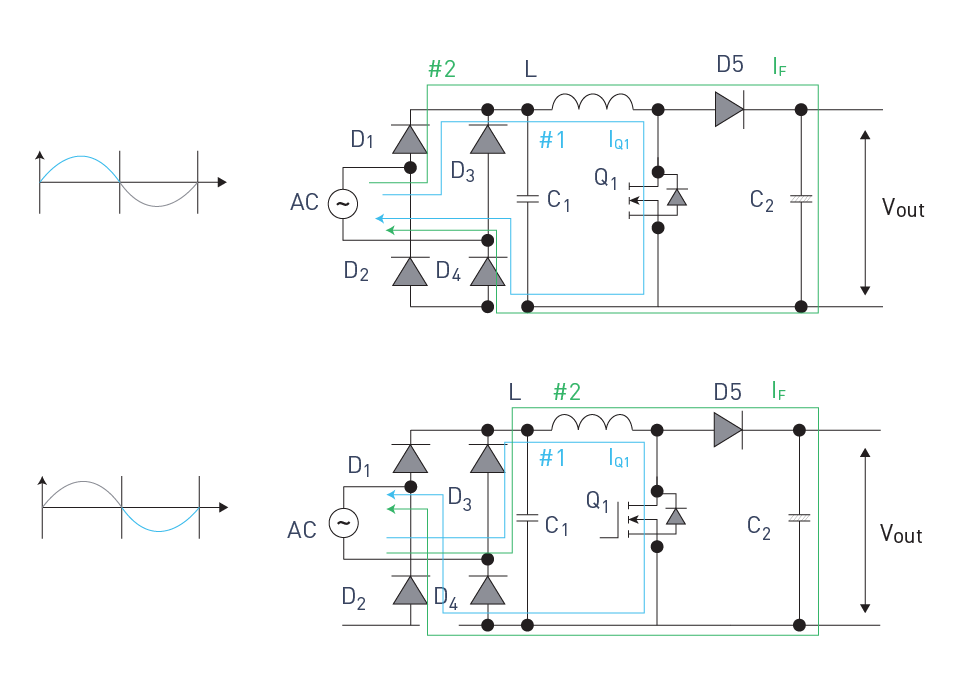
Figure 3: Active PFC circuit with current paths
Active power factor correction (PFC) is more efficient than passive PFC, particularly in conditions with dynamic load. Better power factor correction, decreased heat generation, and a more compact footprint are all features that it offers. Unlike passive PFC, it is more complicated and expensive.
1. Boost Converter-Based PFC:
The active PFC topology is utilized the most frequently. It achieves this by elevating the rectified input voltage to a higher level, subsequently storing it in a bulk capacitor. This helps it function.
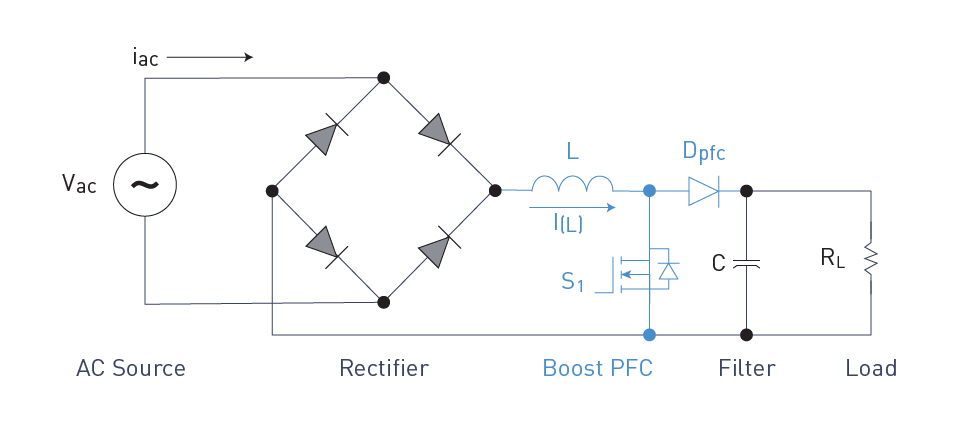
Figure 4: Boost PFC
This topology is most commonly used because of its ease of implementation and efficiency in a wide range of applications, particularly those that require low to medium power loads.
2. Bridgeless PFC:
This design reduces the conduction losses associated with the diodes by eliminating the diode bridge rectifier, which is frequently present in standard PFC circuits.
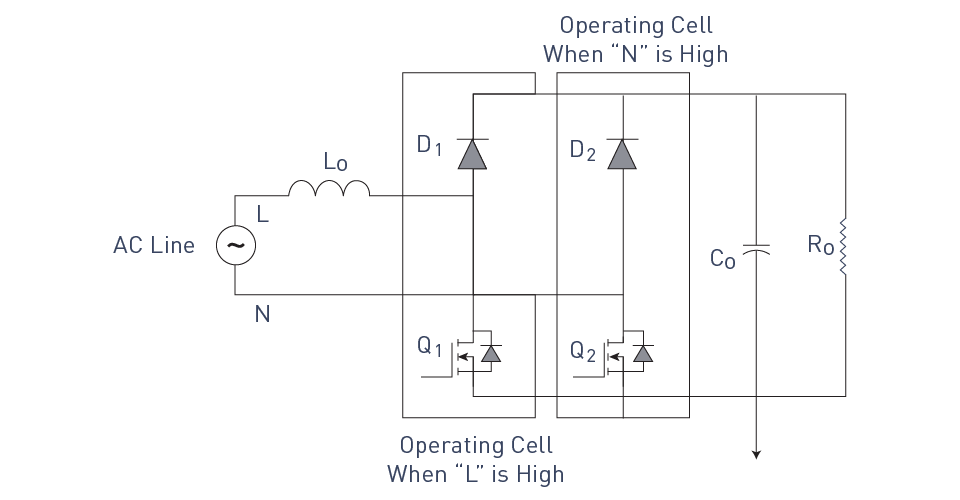
Figure 5: Bridgeless PFC
Its enhanced efficiency makes it suited for high-power applications; however, the design of this product is more complicated than other similar products.
3. Totem-Pole PFC:
The totem-pole power factor converter (PFC) architecture is a variation of the bridgeless power factor converter (PFC) that makes use of a series of transistors that are stacked in a totem-pole arrangement. This configuration reduces switching losses and increases efficiency.
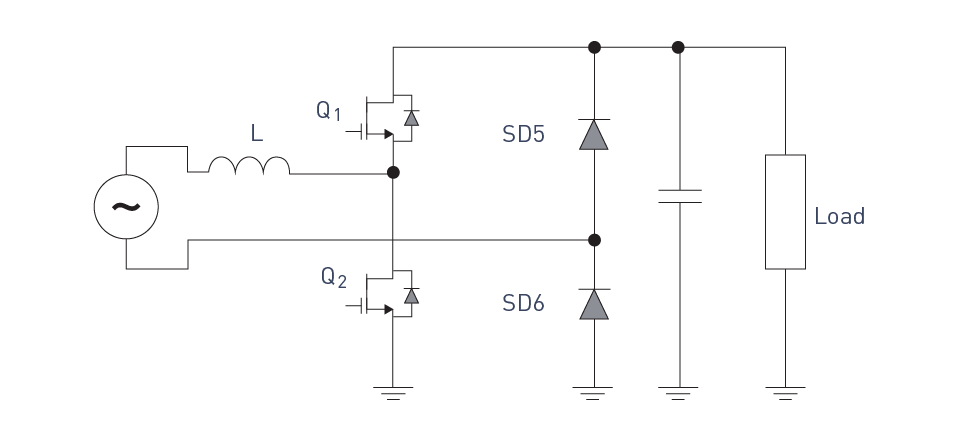
Figure 6: Bridgeless Totem-Pole PFC Schematic
Due to the fact that it is both efficient and small, the topology is becoming increasingly popular in high-performance applications.
4. Interleaved PFC:
The term "interleaved PFC" refers to a configuration in which numerous PFC circuits are functioning in parallel but are not in phase with one another. This interleaving helps to reduce ripple current, which in turn makes it possible to use capacitors and inductors that are smaller and less expensive.
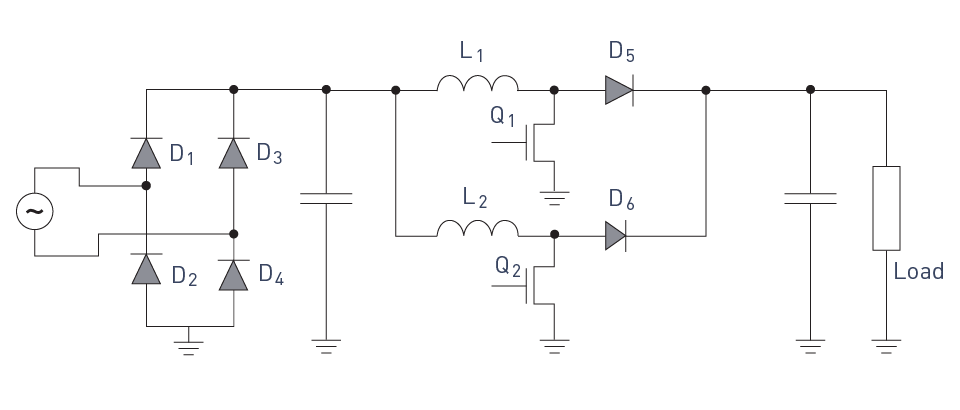
Figure 7: Interleaved Boost PFC Schematic
Interleaved power factor correction (PFC) capacitors offer increased thermal performance and less electromagnetic interference, making them ideal for high-power applications.
PFC controllers come in a variety of types and topologies, each offering a unique set of benefits and tailored to meet specific power requirements and applications. A number of considerations, including efficiency, cost, size, and the particular power requirements of the application are taken into consideration when making the decision between passive and active power factor converters (PFC), as well as the selection of a specific active PFC topology.
Applications of Active Power Factor Correction
Variable Load Equipment: Active power factor correction (PFC) is very useful for machinery that has variable loads, such as variable speed drives, because it allows for a rapid change in the amount of reactive power that is required. Active power factor correction (PFC) has the ability to adjust to these changes, thereby delivering accurate compensation and preserving a high power factor despite the varied operating conditions.
Data Centers and IT Infrastructure: Power supplies of servers, networking equipment, and other types of information technology infrastructure frequently incorporate active power factor correction (PFC) to reduce electrical losses, reduce heat generation, and increase the dependability of PSUs.
Renewable Energy Systems: In renewable energy systems, active power factor correction (PFC) is utilized in solar inverters and wind turbine converters to guarantee that the power that is sent into the grid is of a quality that satisfies quality requirements, the correction of power factor, and the reduction of harmonic distortion.
Industrial Automation: Active power factor correction (PFC) is beneficial to complex industrial processes that involve robotics, automation, and precision machinery because it maintains constant power supply characteristics, even when the load varies rapidly while the process is being carried out.
Implications of Active Power Factor Correction
Enhanced Efficiency: Active power factor correction (PFC) is a technique that dramatically minimizes energy losses in the system, which ultimately results in an improvement in overall efficiency. This is accomplished by adjusting the power factor to be as close to unity as feasible. This increase in efficiency results in a reduction in both the amount of energy consumed and the costs of operations.
Improved Power Quality: Active power factor correction (PFC) systems have the ability to improve power quality by addressing not only power factor concerns but also other power quality issues, such as harmonic distortion. The stability and quality of the electrical supply are improved as a result of their actions, which is essential for sensitive electronic equipment and adds to the longevity of the electrical infrastructure.
Dynamic Adaptability: Active power factor correction (PFC) is extremely adaptable to a wide variety of applications due to its dynamic adaptability, which is the capacity to respond in real time to changes in the load or supply circumstances. This flexibility is especially significant in modern power systems, which are increasingly characterized by variable renewable energy sources and varying demand patterns. This flexibility is particularly important in modern power systems.
Economic and Regulatory Compliance: The deployment of active power factor correction (PFC) assists enterprises in meeting regulatory standards for power quality and efficiency, which may allow them to avoid penalties for having a power factor that is below average. Additionally, active PFC has the potential to give significant economic benefits over time by lowering the amount of energy that is lost and the demand charges that are incurred on utility bills.
Technical Complexity and Cost: When compared to passive approaches, active photovoltaic solar cells (PFC) have a higher starting cost and a higher level of technological complexity. These are the primary problems that are linked with active PFC. Active power factor correction (PFC) systems require specialist understanding in power electronics and control systems in order to be designed, implemented, and maintained.
Hybrid Power Factor Correction
In order to maximize the power quality and efficiency of alternating current (AC) power systems, hybrid power factor correction (PFC) systems are an innovative solution that incorporates the advantages of both passive and active power factor correction methodologies. Through the utilization of passive parts, which are known for their simplicity and dependability, this clever technique takes advantage of the flexibility and accuracy that active controls offer. Enhanced corrective capabilities and dynamic adaptation to variable load conditions are both made possible by the integration of these technologies into a cohesive system. The hybrid power factor correction concept and its implications are examined in this section.
Concept and Functionality
Generally speaking, hybrid power factor correction (PFC) systems consist of two stages: an active circuit or PFC stage that uses power electronics to dynamically adjust the compensation based on the system's conditions in real time, and a passive PFC stage that may include fixed or automatically switched capacitors.
One example of hybrid power factor correction is the utilization of passive power factor correction (PFC) for the management of reactive power and active harmonic filtration for the management of system harmonics.
Passive and active power factor correction techniques can also be combined in hybrid power factor correction. For instance, a hybrid power factor correction (PFC) system can make use of passive PFC for stable, static operation and active PFC for transient responses, harmonic distortion, and changes in the amount of reactive power that is required. The use of active PFC is minimized, which helps to lower the amount of heat that is generated, which is one of the benefits of this technique.
Applications of Hybrid Power Factor Correction
Industrial Facilities: In settings that include a combination of constant and variable loads, such as industrial plants, hybrid power factor correction (PFC) is able to effectively handle the various power quality needs, ensuring that machinery and equipment are able to function at their highest potential.
Commercial Buildings: The use of hybrid systems is most suited for commercial settings that have dynamic electrical loads. These settings include office buildings and retail centers, where hybrid systems contribute to the reduction of energy costs and the improvement of the reliability of the power supply.
Utility Networks: Utility providers have the ability to use hybrid power factor correction (PFC) systems on a greater scale in order to increase grid stability, more effectively manage peak demands, and facilitate the integration of renewable energy sources.
Implications of Hybrid Power Factor Correction
Enhanced Efficiency and Reliability: It is possible for hybrid systems to achieve higher degrees of power factor correction and power quality improvement by combining passive and active correction approaches. This results in better system efficiency and dependability.
Cost-Effectiveness: When compared to solely active systems, hybrid power factor correction (PFC) systems have the potential to provide a more cost-effective solution. This is particularly true in situations where a major percentage of the correction may be accomplished through the use of passive components that are less expensive.
Flexibility and Scalability: The modular nature of hybrid systems enables scalability and adaptation to specific application needs and future growth, which makes them a versatile solution for a wide range of scenarios. Scalability and adaptability are also allowed by hybrid systems.
Complexity and Integration Challenges: Hybrid systems, despite the fact that they offer a multitude of advantages, also present additional challenges in terms of design, integration, and maintenance. In order to maximize the benefits and minimize the potential problems, it is essential to build and administer the system in an appropriate manner.
Regulatory and Environmental Benefits: Hybrid power factor correction (PFC) systems can assist enterprises in meeting regulatory criteria and contributing to environmental sustainability efforts by decreasing energy consumption and greenhouse gas emissions. The system achieves this by improving the power factor and reducing reactive power flows.
直接登录
创建新帐号