Infrastructure Investment Decisions
Ensuring a dependable, effective, and sustainable energy supply requires long-term planning and investment analysis in electrical systems. This entails choosing wisely when it comes to increasing generating capacity and improving transmission and distribution networks. In order to incorporate new technology, meet future demand, and take social and environmental concerns into account, these expenditures are needed.
Generation Capacity Expansion
Assessing Future Demand: Precise demand forecasting is the first step towards increasing generating capacity. Factors like population expansion, economic development, technological improvements, and patterns of energy usage are all important considerations for planners. Utilities can forecast future power demand and calculate the additional generation capacity needed by examining these parameters.
Resource Availability and Diversity: Increasing generation capacity entails assessing the potential and availability of a range of energy sources, including nuclear power, fossil fuels, and renewables like biomass, solar, wind, and hydropower. A varied energy portfolio lowers reliance on any one resource, improves system resilience, and lessens the risk of supply disruptions and volatile fuel prices.
Technology Selection and Siting: For effective and long-term growth, the right generating technologies must be chosen. A few things to think about include the cost and maturity of the technology, site availability, environmental impact, and efficiency. For example, while fossil fuel plants could need to be close to fuel sources and water for cooling, renewable energy projects might need particular geographic and climatic conditions.
Economic and Environmental Considerations: The Levelized Cost of Electricity (LCOE), which takes into account plant lifetime maintenance, operating, and capital expenses, is a key component in determining the economic viability of new generation projects. Assessments of the effects on the environment, including greenhouse gas emissions, land use, and water consumption, are also necessary. Reducing environmental footprints and adhering to regulations need investments in cleaner energy sources and low-carbon technologies.
Regulatory and Policy Frameworks: Government rules and policies have a big impact on increasing generation capacity. Investment in specific technologies can be encouraged by incentives like tax credits, subsidies, and renewable energy standards. In order to match their strategies with regional and national energy policies, planners need to stay up-to-date on regulatory developments.
Transmission and Distribution Infrastructure
Enhancing Grid Capacity and Reliability: In order to manage growing loads and preserve dependability, the transmission and distribution (T&D) infrastructure must be modernized in tandem with the expansion of generation capacity. This entails building new lines, modernizing substations, and strengthening already-existing transmission lines. Stable power supply to end customers is guaranteed by a strong transmission and distribution network, which also avoids bottlenecks and transmission losses.
Integrating Renewable Energy Sources: The current grid infrastructure has difficulties when integrating variable renewable energy sources like solar and wind. To control the erratic and unpredictable nature of renewable energy, investments must be made in cutting-edge grid technology like Flexible AC Transmission Systems (FACTS), High Voltage Direct Current (HVDC) lines, and smart grid technologies. These technologies improve the efficiency, stability, and flexibility of the grid.
Smart Grid Technologies: Modernizing the grid requires spending money on smart grid technologies, such as automated control systems, real-time monitoring, and sophisticated metering infrastructure. These technologies make it easier to integrate dispersed energy resources, enhance demand response, and manage outages. Smart grids improve the electrical system's overall dependability and efficiency.
Addressing Aging Infrastructure: An aging T&D infrastructure can result in greater maintenance costs, a higher failure rate, and decreased reliability. This is a problem that many regions encounter. Plans for the long term must address how to update or replace antiquated machinery and systems. Investments in modernization made proactively can enhance system performance and avert expensive problems.
Regulatory and Environmental Compliance: Investments in infrastructure must abide by all applicable laws and environmental guidelines. This entails completing environmental impact assessments, securing the required permits, and abiding by zoning and land use laws. Social effects, including possible relocation and community acceptance, must also be taken into account by planners.
Financial and Economic Analysis: Financing T&D infrastructure projects requires an extensive economic study that includes risk assessments and cost-benefit analyses. Funding for planners needs to come from a variety of places, such as public-private partnerships, private investments, and government subsidies. Infrastructure projects are guaranteed to be feasible and sustainable through transparent and prudent financial planning.
Demand Forecasting and Scenario Analysis
Precise demand forecasts and thorough scenario analysis are essential for long-term planning and investment in electrical systems. Utility firms and grid operators may predict future electricity needs, evaluate different possible developments, and make well-informed decisions on infrastructure expenditures thanks to these methods.
Demand Forecasting
Importance of Demand Forecasting
The practice of projecting future power consumption using past data, present trends, and expected shifts in affecting factors is known as demand forecasting. To guarantee that there is enough infrastructure and generating capacity to safely and economically fulfill future demand, accurate projections are essential.
Methods of Demand Forecasting
Historical Data Analysis: This technique looks at historical consumption trends to find seasonality and trends. Regression models and time series analysis are two statistical methods that are frequently used to forecast future demand from historical data.
Econometric Models: These models anticipate electricity consumption by including economic factors such as changes in population, GDP growth, and industrial production. Through an understanding of the correlation between economic variables and energy use, planners are able to forecast the impact of future economic fluctuations on demand.
End-Use Analysis: This method entails looking at the particular consumption trends of various industries, including commercial, industrial, agricultural, and residential. Planners can create more precise and detailed projections by examining the elements influencing demand in each industry.
Artificial Intelligence and Machine Learning: Sophisticated methods such as neural networks and machine learning algorithms are increasingly crucial for demand forecasting. Large datasets and intricate interactions can be handled by these techniques, which result in more precise and dynamic forecasts.
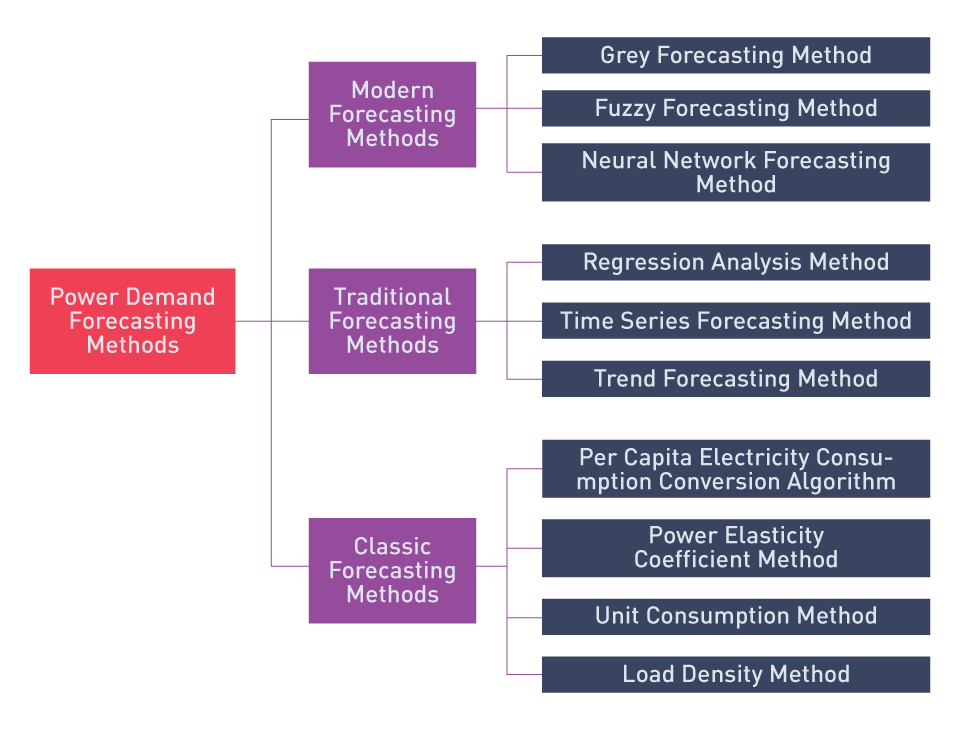
Figure 9: Power demand forecasting methods
Challenges in Demand Forecasting
Data Quality and Availability: Comprehensive, high-quality data is necessary for accurate forecasting. Data gaps, measurement flaws, and out-of-date information can all affect how accurate a forecast is.
Uncertainty and Variability: Demand estimates are subject to uncertainty and unpredictability due to various factors, including legislative changes, technical improvements, and alterations in consumer behavior. Addressing these concerns can be aided by scenario analysis.
Scenario Analysis
Purpose of Scenario Analysis
Analyzing various future developments and their effects on infrastructure requirements and electricity demand is known as scenario analysis. Planners may create adaptable, strong strategies and gain a better understanding of the possible risks and possibilities by taking into account a variety of scenarios.
Developing Scenarios
Baseline Scenario: This scenario is predicated on the continuation of present practices and laws without much alteration. It offers a benchmark against which different situations might be evaluated.
High Growth Scenario: This scenario takes into account faster-than-anticipated economic development, more electrification, and quick adoption of new technologies, all of which raise the demand for power.
Low Growth Scenario: This scenario predicts lower demand for electricity due to slower economic development, greater energy efficiency, and maybe a decline in population.
Policy-Driven Scenario: This scenario looks at the effects of new laws, financial aid, and incentives that support decarbonization, renewable energy, and energy efficiency. It aids in evaluating the possible impacts of policy modifications on demand and infrastructure requirements.
Technological Change Scenario: This scenario investigates the effects of major technological developments, including distributed generation, smart grid technology, and the widespread use of electric vehicles. It aids in planners' comprehension of how new technologies could change demand trends and infrastructure needs.
Analyzing Scenarios
Impact Assessment: The assessment of each scenario's possible influence on the demand for electricity, the capacity of generation, and the requirements for infrastructure is done. This entails both qualitative evaluations of potential dangers and possibilities and quantitative analysis utilizing models and simulations.
Strategic Planning: Using the results of the scenario analysis, planners create plans to take advantage of the opportunities and deal with any obstacles that may arise. This could involve making investments in additional generation capacity, improving the infrastructure for transmission and distribution, and launching programs to improve demand response and energy efficiency.
Flexibility and Adaptability: Using scenario analysis, planners may create strategies that are adaptive and flexible enough to be modified in response to real-world events. Utilities can assure dependable, economical service and more effectively manage uncertainty by planning for a variety of potential futures.
Cost-Benefit Analysis for Investments
To guarantee that resources are distributed effectively and sustainably, comprehensive cost-benefit analysis is necessary for long-term planning and investment in electrical systems. This section examines three essential elements of cost-benefit analysis, namely the Levelized Cost of Electricity (LCOE), System Reliability and Risk Assessment, and Environmental and Social Costs, in relation to investments in the electricity system.
Levelized Cost of Electricity (LCOE)
Definition and Importance: Considering all expenses, the levelized cost of energy (LCOE) is the average cost per unit of energy produced during a power plant's lifetime. For evaluating the financial sustainability of various energy generation systems and directing investment choices, life cycle costs (LCOE) are an essential measure.
Calculation of LCOE: The following formula is used to compute LCOE:
$$\text{LCOE} = \frac{\text{NPV of Total Costs over Lifetime}}{\text{NPV of Electrical Energy Produced over Lifetime}} = \frac{\sum \frac{I_t + M_t + F_t}{(1 + r)^t}}{\sum \frac{E_t}{(1 + r)^t}}$$Where:
NPV = Net Present Value, the value of all future negative and positive cash flows over the entire lifespan of an investment discounted to the present
\( I_t \)= Investment costs in year
\( M_t \)= Operations and maintenance costs in year
\( F_t \)= Fuel costs in year
\( E_t \)= Electricity generated in year
\( _r \)= Discount rate of the project
\( _t \)= Year
This computation includes the estimated production of the plant over its operational life, adjusted for time value of money, along with capital costs, fuel costs, and operating and maintenance expenses.
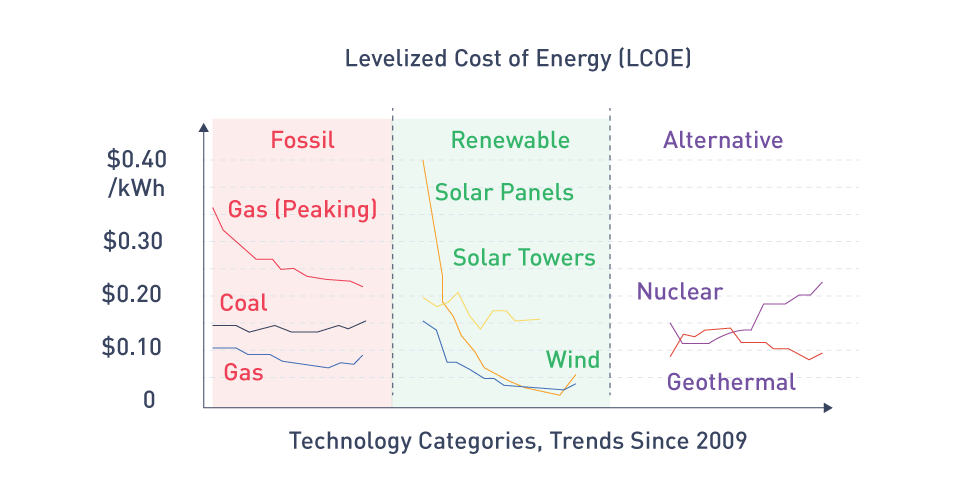
Figure 10: LCOE comparison for different generation methods
Application of LCOE: Using LCOE, one may compare various energy sources, including coal, natural gas, nuclear, wind, and solar. More economical generating options are indicated by lower LCOE values. Policymakers and investors can prioritize projects that deliver the best economic return while taking other considerations like environmental impact and energy security into account by comparing the Life Cycle Cost of Equity (LCOE) across different technologies.
System Reliability and Risk Assessment
Importance of System Reliability
System dependability, which guarantees a steady supply of power that is resistant to interruptions, is a crucial factor in the planning of electrical systems. Systems that are dependable reduce downtime, avert blackouts, and preserve stability in the face of fluctuating demand and possible malfunctions.
Reliability Metrics and Models
Loss of Load Probability (LOLP): This gauges the likelihood that the system won't be able to satisfy demand at any one moment.
Expected Energy Not Supplied (EENS): Calculates the total energy demand that is unmet as a result of inadequate system performance.
N-1 Criterion: The N-1 criterion guarantees that a single component failure won't cause the system to go down and cause widespread disruptions.
Risk Assessment
Risk assessment includes locating possible threats to the dependability of the system, like malfunctioning equipment, natural disasters, cyberattacks, and the incorporation of renewable energy sources. Techniques like Monte Carlo simulations and probabilistic risk assessment (PRA) can quantify risks and assess mitigation measures.
Mitigation Strategies
Redundancy: To improve resilience, build backup and redundant systems.
Preventive Maintenance: This involves routine inspections and repairs to avoid unexpected malfunctions.
Advanced Grid Management: Putting smart grid technology into practice for dynamic disruption response and real-time monitoring.
Environmental and Social Costs
Environmental Impact: The production of electricity and the construction of infrastructure have a substantial negative influence on the environment. These effects include the release of greenhouse gasses into the atmosphere, contamination of the air and water, destruction of habitats, and depletion of resources. It is essential for sustainable development to assess these effects.
Social Costs: These are the wider ramifications of power investments in the community, including changes in employment patterns, pollution-related health effects, and relocations brought on by infrastructure projects. Community involvement and social acceptance are essential to the effective execution of energy projects.
Valuation of Environmental and Social Costs:
Carbon Pricing: To account for the impact on the climate, carbon emissions are given a monetary value.
Life Cycle Analysis (LCA): The Life Cycle Analysis (LCA) method evaluates a project's overall environmental impact from the point of development to the point of decommissioning.
Social Cost-Benefit Analysis: including externalities and non-market values in project economic assessments.
Integrating Environmental and Social Costs into Decision Making: Setting priorities and making trade-offs are necessary to balance economic, environmental, and social factors. Using multi-criteria decision analysis (MCDA) and integrated assessment models, policymakers and investors can assess many situations and find solutions that optimize total benefits while reducing negative effects.
直接登录
创建新帐号