Wireless Power Transfer
Wireless power transfer (WPT) is a breakthrough technology in electrical engineering that allows electrical energy to be transmitted without the need for physical connectors. WPT is mostly used with two methods: capacitive and inductive. Short-distance, low-power applications can benefit from capacitive power transfer. Inductive power transfer can utilize larger air gaps of several meters, resulting in a relatively higher output power.
Principles and Applications of Inductive Wireless Power Transfer
Electromagnetic induction is used in inductive wireless power transmission to transfer power between a transmitter and a receiver coil. A voltage is induced in the reception coil when an alternating current (AC) passes through the transmitter coil, producing a fluctuating magnetic field. Batteries can then be charged or electrical equipment can be powered by this induced voltage.
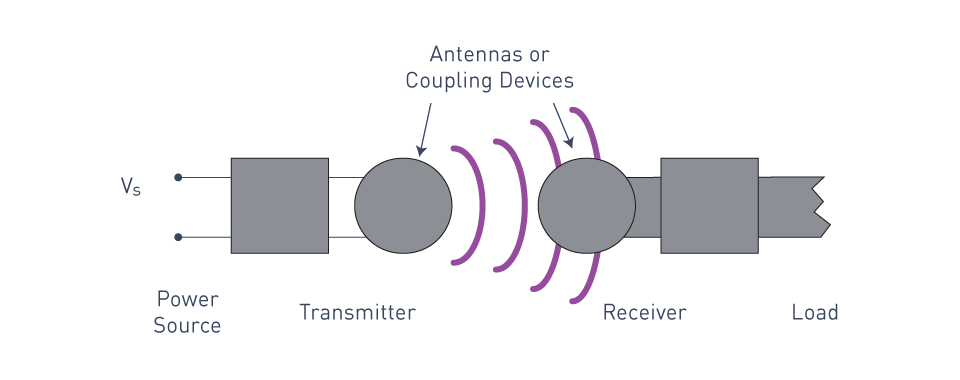
Figure 6: Generic wireless power system
Principles: The coupling coefficient, which is impacted by the coils' alignment, spacing, and individual geometries, determines how efficient inductive power transfer is. Resonant inductive coupling increases the range and efficiency of power transfer when both coils are tuned to the same resonant frequency.
Applications: Wearable technology and wireless charging pads for cellphones are only two examples of consumer electronics that make extensive use of inductive WPT. Additionally, it is used in EV charging systems, which enable EVs to park over charging pads and charge wirelessly. Industrial uses include supplying energy to remote sensors in dangerous locations where conventional cable is problematic and powering medical implants like pacemakers.
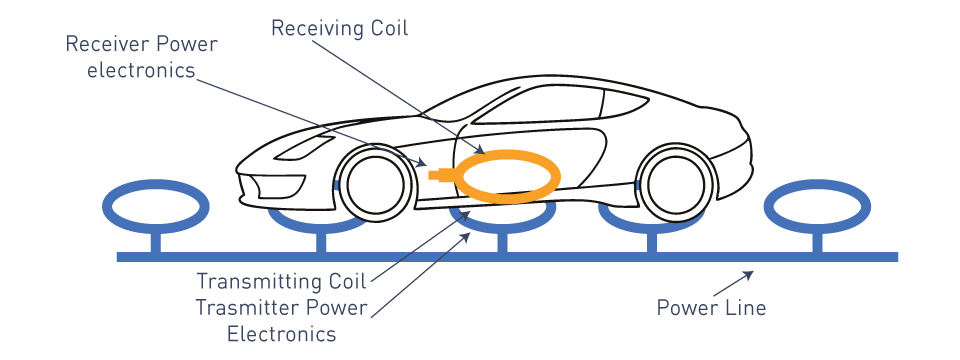
Figure 7: Wireless EV charging
Principles and Applications of Capacitive Wireless Power Transfer
Electric fields are used in capacitive wireless power transfer to move energy between two conducting plates, or electrodes. Through the dielectric material separating them, an oscillating electric field produced by an AC voltage delivered to the transmitter plate causes a displacement current to be induced in the receiver plate.
Principles: The area of the plates, their distance from one another, and the dielectric characteristics of the medium all affect how efficient capacitive power transfer is. High-frequency operation and proper alignment can greatly improve the power transfer capacity.
Applications: Where inductive WPT is less successful, such as through conductive materials or in situations where coil alignment is difficult, capacitive WPT is employed. It can power sensors, RFID systems, and some medical equipment, among other low-power uses. Researchers are also investigating capacitive coupling for wireless power transfer to consumer electronics and to create effective charging systems for small, portable gadgets.
Potential Impact on Consumer Electronics, Electric Vehicles, and Industrial Applications
Consumer Electronics: Wireless power transfer has completely transformed the way we charge and power our electronics. The user experience has been greatly enhanced by the ease with which a device may be placed on a charging pad without the need for cords. Future developments in WPT technology should make it possible to charge over longer distances and even through surfaces, facilitating the more seamless integration of power solutions in homes and offices. Since gadgets no longer need to include charging ports, the use of WPT in consumer electronics also opens the door for novel form factors and designs.
Electric Vehicles: By using inductive WPT, a number of issues with traditional plug-in charging systems are resolved for electric vehicles. Wireless EV charging improves user convenience and safety by removing the need for physical connectors and wires, reducing wear and tear, and lowering the possibility of electric shock. Vehicles that use dynamic wireless charging, in which they charge while traveling on specially designed highways, may be able to increase their driving range and require smaller batteries. The development of wireless EV charging infrastructure has the potential to significantly accelerate the shift to electric vehicles.
Industrial Applications: Wireless power transfer has several benefits in industrial environments, such as lower maintenance costs and increased safety. WPT can power equipment in places like chemical facilities, underwater installations, or areas with a lot of dust and debris where conventional wire is unsafe or unfeasible. When autonomous robots and drones are wirelessly charged, they may operate continuously without needing human intervention, which increases operational efficiency. WPT can also make it easier to install sensors and monitoring equipment in hard-to-reach places, which will help the Industrial Internet of Things (IIoT) expand.
Energy Storage Solutions
Role of Energy Storage in Stabilizing AC Power Systems
Energy storage solutions are essential for maintaining the stability of AC power networks since they act as a buffer, able to store surplus energy and release it when needed. Maintaining the equilibrium between electricity supply and demand is dependent on this skill, particularly with the growing integration of intermittent renewable energy sources like wind and solar power.
Key Functions of Energy Storage in AC Power Systems:
Frequency Regulation: Energy storage systems (ESS) have the ability to quickly inject or absorb power in response to variations in power frequency. This contributes to keeping the grid frequency within reasonable bounds, guaranteeing steady and dependable functioning.
Peak Shaving: Energy storage can release stored energy to lighten the burden on the grid during times of high electricity demand. Peak shaving is a technique that can help to reduce strain on the electrical grid and postpone the need for expensive transmission and distribution network expansions.
Load Balancing: ESS has the capacity to store extra energy produced when demand is low and release it when demand is at its highest. This load balancing capacity makes it easier to manage the fluctuation of renewable energy sources, which occasionally produce more power than needed and sometimes less.
Voltage Support: Energy storage can assist in keeping voltage levels within the intended range by supplying reactive power. This helps to ensure that electrical equipment operates smoothly and lowers the possibility of voltage spikes or sags.
Black Start Capability: Energy storage systems can supply the first electricity required to restart power plants and resume regular grid operations in the case of a significant grid loss, providing an essential backup in an emergency.
Advances in Battery Technology
Energy storage devices now function, operate, and are much more affordable thanks to advancements in battery technology. Grid storage now uses a variety of battery types, each with special qualities of its own:
Lithium-Ion Batteries:
Advantages: A long cycle life, a high energy density, and quick response times.
Applications: Frequently utilized in portable electronics, electric cars, and grid storage.
Advancements: Through recycling and the use of alternative materials, ongoing research aims to increase the sustainability, lower costs, improve safety, and improve the energy density of lithium-ion batteries.
Sodium-Sulfur (NaS) Batteries:
Advantages: Long discharge times and a high energy density.
Applications: Fit for large scale energy storage uses.
Advancements: To increase the dependability and effectiveness of NaS batteries, efforts are being made to extend their lifespan and expand their operating temperature range.
Flow Batteries:
Advantages: Scalability, a long cycle life, and the capacity to supply energy for longer periods of time are advantages.
Applications: Perfect for long-term, large-scale energy storage applications.
Advancements: Research is concentrated on creating electrolytes that are more economical and efficient while also refining the system's general architecture to lower maintenance needs.
Other Storage Methods
Flywheel Energy Storage Systems (FESS):
Principle: Flywheels function by rapidly rotating a rotor to store kinetic energy. The rotor is slowed down to release the energy as electrical power.
Advantages: A high power density, quick reaction times, and a long lifespan with little deterioration.
Applications: Perfect for controlling frequency and meeting temporary storage requirements.
Advancements: Flywheel systems are becoming more efficient, energy dense, and economically viable due to advancements in materials and design.
Supercapacitors:
Principle: Supercapacitors have quick charge and discharge times and store energy via electrostatic charge separation.
Advantages: A long cycle life, quick response time, and high power density.
Applications: Useful for tasks like voltage stability and bridging power gaps that call for brief power surges.
Advancements: The objective is to reduce costs and boost energy density, enabling the use of supercapacitors in a broader range of applications.
Compressed Air Energy Storage (CAES):
Principle: When electricity is required, CAES devices store energy by compressing air and releasing it to power a turbine.
Advantages: Long duration and large storage capacity.
Applications: Suitable for load leveling and large-scale energy storage.
Advancements: The overall efficacy of CAES technology is being enhanced by improvements in system efficiency and the creation of adiabatic CAES, which collects and repurposes heat produced during compression.
Microgrids and Distributed Generation
Concept and Implementation of Microgrids
A microgrid is a small collection of electrical sources and loads that can function separately as an island system or in tandem with the larger grid. Enhancing the sustainability, efficiency, and dependability of the energy supply within a designated area is the core idea of a microgrid. Microgrids combine energy storage technology and demand management with a variety of distributed generation (DG) sources, including wind turbines, solar panels, and combined heat and power (CHP) units.
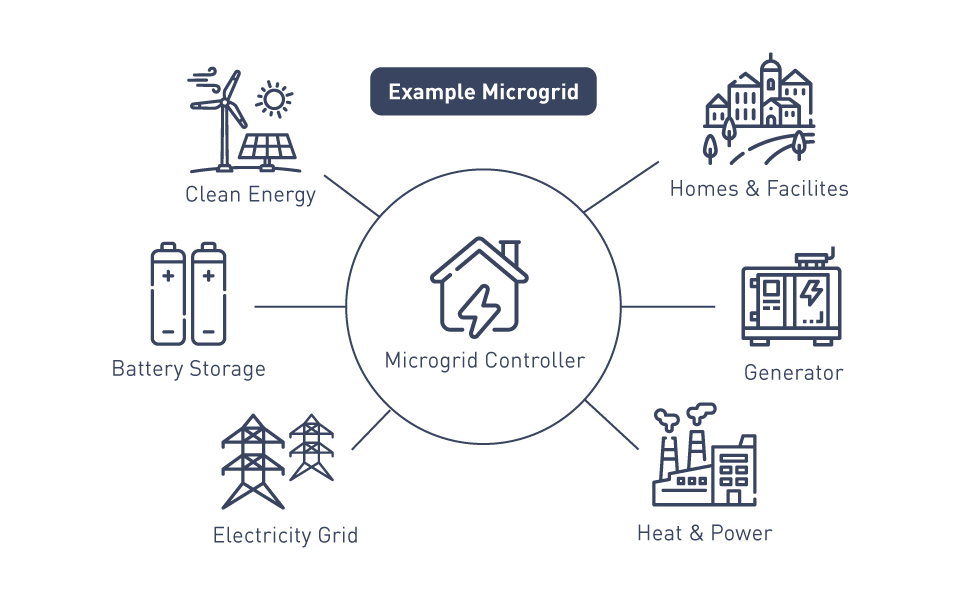
Figure 8: Microgrid concept
Key Components and Features:
Distributed Generation Sources: To offer local generation and lessen reliance on centralized power plants, microgrids often integrate a number of DG sources. These sources might be conventional generators like natural gas or diesel units, or they can be renewable energy sources like solar photovoltaic (PV) panels and wind turbines.
Energy Storage Systems: When using intermittent renewable energy sources, it is especially important to balance supply and demand by using energy storage devices like flywheels and batteries. The microgrid's stability and dependability are increased by storage devices, which enable it to continue supplying electricity even in the event of fluctuations in generation.
Control Systems: To oversee the functioning of microgrids, sophisticated control systems are necessary. To ensure optimal performance, these systems coordinate the production, storage, and consumption of power. They allow the microgrid to react dynamically to variations in load or generation and to smoothly transition between grid-connected and islanded modes.
Load Management: To balance available supply and demand, microgrids use load management strategies. Demand response systems are important for improving energy use in microgrids because they modify consumption in response to price and availability signals.
Grid Interconnection: Microgrids can import or export power as needed when they are linked to the main grid. When switching between islanded and grid-connected modes, safe and dependable operation is ensured via grid connectivity standards and protocols.
Implementation Process:
Feasibility Study: The first stage in putting a microgrid into operation is to carry out an extensive feasibility assessment. This includes evaluating the project's economic feasibility, resource availability, and energy requirements.
Design and Engineering: Extensive design and engineering work is required to establish the microgrid's components, configuration, and control schemes. This entails picking suitable control systems, storage options, and generating sources.
Construction and Installation: Setting up the microgrid entails setting up the control infrastructure, connectivity hardware, generation and storage devices. The microgrid's integration with the current electrical infrastructure is another aspect of this phase.
Testing and Commissioning: To guarantee that every component operates accurately and effectively, the microgrid must go through extensive testing and commissioning prior to being put into full operation. This stage confirms that the microgrid can function in both islanded and grid-connected modes.
Operation and Maintenance: Continuous operation and maintenance are essential to the microgrid's long-term viability. Ongoing maintenance guarantees peak performance, and operational plans adjust to shifting circumstances and energy requirements.
Enhancing Grid Resilience
In order to improve grid resilience and encourage the incorporation of renewable energy sources, microgrids are essential. Even in the event of main grid failures, microgrids can deliver a steady and dependable power supply by localizing, generating, and integrating energy storage.
Islanded Operation: Microgrids' capacity to function separately from the main grid is one of its main benefits. Microgrids can easily switch to an islanded state during a grid outage, preserving electricity for essential loads and boosting the energy system's overall resilience.
Black Start Capability: Because of their black start capability, microgrids have the ability to restart local generating sources independently of the main grid. This feature is necessary to promptly restore electricity following a broad outage.
Distributed Energy Resources: Microgrids lessen the sensitivity of the energy system to localized interruptions by dispersing generation sources among several sites. The decentralization process improves the grid's resilience and dependability.
Promoting Renewable Integration
Local Utilization of Renewables: Microgrids lower transmission losses and increase the energy system's efficiency by enabling the local production and use of renewable energy. This strategy maximizes the renewable resources that are available locally.
Energy Storage Integration: Adding energy storage components to microgrids reduces the use of intermittent renewable energy sources like solar and wind power. Storage systems guarantee a consistent and dependable power supply by storing excess energy produced during peak production times and releasing it during low production times.
Grid Support Services: By controlling the production and consumption of power in real-time, microgrids can provide important grid support services, including voltage support and frequency management. These services facilitate the integration of higher amounts of renewable energy and enhance the stability and dependability of the larger grid.
Pilot Projects and Innovation: Microgrids function as experimental platforms for novel technologies and strategies for integrating renewable energy sources. Microgrids aid in the creation of best practices and scalable strategies for wider adoption by testing and improving novel ideas on a smaller scale.
Artificial Intelligence and Machine Learning in Power Systems
The fields of artificial intelligence (AI) and machine learning (ML) are transforming AC power systems by bringing cutting-edge capabilities to problem detection, predictive maintenance, and grid optimization. These technologies improve power systems' resilience, efficiency, and dependability by utilizing enormous volumes of data and complex algorithms.
Applications of AI and ML for Predictive Maintenance
Predictive maintenance is the process of using AI and ML to evaluate data from power system components in order to forecast faults before they happen. By taking a proactive stance, utilities can cut downtime and maintenance costs by only performing services when absolutely essential, as opposed to following a set schedule.
Data Collection and Analysis:
Data on the operational parameters of transformers, circuit breakers, and generators is gathered by sensors and monitoring devices. Temperature, vibration, load, and other performance metrics are included in this data.
- AI algorithms analyze this data to find trends and abnormalities that point to possible problems.
Fault Prediction Models:
- Machine learning models are developed using historical data in order to identify early indications of upcoming failures and component degradation.
- Over time, these models gain greater and more predicted accuracy as they process and analyze more data.
Maintenance Scheduling:
- Based on the data, predictive maintenance systems provide alerts and suggestions for maintenance tasks.
- This method ensures the precise execution of maintenance tasks when necessary, thereby reducing interference and enhancing the longevity of equipment.
Applications of AI and ML for Grid Optimization
AI and ML are essential for improving the efficiency, stability, and flexibility of the electrical grid, which in turn optimizes its functioning. These technologies enable real-time decision-making and adaptive control techniques that dynamically adapt to changing situations.
Load Forecasting:
- Precise load forecasting is necessary to maintain equilibrium between supply and demand. AI models forecast future electricity demand with a high degree of accuracy by examining past consumption trends, meteorological data, and other pertinent variables.
- Enhanced load forecasts facilitate more efficient planning and resource allocation by grid operators, lowering the risk of blackouts and maximizing the utilization of power assets.
Energy Management:
- Artificial intelligence (AI)-driven energy management systems maximize the performance of distributed energy resources (DERs), such as solar panels and battery storage, by balancing their output to effectively meet demand.
- These systems dynamically modify storage and generation to save expenses, cut emissions, and improve grid stability in general.
Voltage and Frequency Regulation:
- AI algorithms oversee and regulate voltage and frequency levels throughout the grid, guaranteeing they stay within reasonable bounds.
- When renewable energy generation is highly variable, advanced control algorithms alter the output of generators and other devices in real-time to stabilize the grid.
Applications of AI and ML for Fault Detection
Effective fault management and detection are essential to preserving power systems' dependability and security. AI and ML allow for rapid identification and diagnosis of problems, which improves fault detection and facilitates prompt mitigation and action.
Anomaly Detection:
- Machine learning models examine data in real-time from sensors and monitoring apparatus to find irregularities that might point to problems.
- These models can detect subtle departures from standard operating conditions that conventional approaches would overlook.
Fault Classification:
- AI systems categorize defects based on their features and consequences. This classification aids in resource allocation and response action prioritization.
- To precisely discern between various failure kinds and their causes, fault classification models are trained using past fault data.
Response and Restoration:
- Decision support systems powered by AI help grid operators create and carry out response plans. These systems offer suggestions for rerouting electricity, isolating the issue, and resuming operation.
- Automated response systems have the ability to promptly execute remedial measures, diminishing the length and severity of disruptions.
直接登录
创建新帐号