Wireless Battery Management Systems
Introduction to Wireless BMS
The evolution of electric mobility and renewable energy storage has led to exciting advancements in battery management technology. One such development in this field is the emergence of Wireless Battery Management Systems (wBMS). Unlike traditional wired BMS, which rely on physical connections to monitor and control individual battery cells, wBMS makes use of wireless communication protocols to interact with these cells.
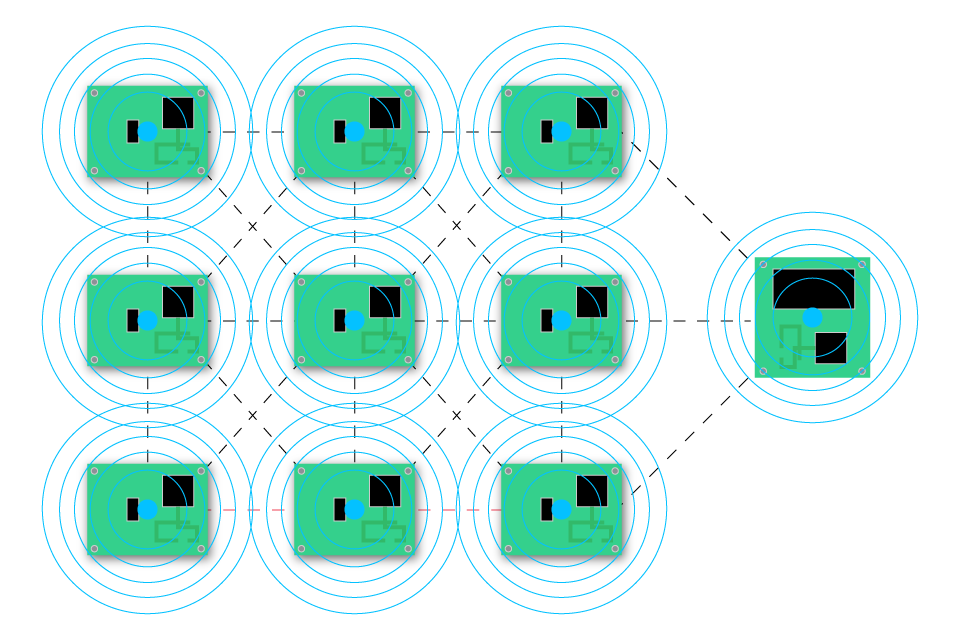
Figure 2: Wireless mesh network for BMS
At the core of a wBMS is a wireless control unit, often accompanied by a sensor module attached to each battery cell. These sensor modules wirelessly transmit data related to the condition of each cell, including information such as voltage, temperature, and current, to the central control unit. The control unit utilizes this data to effectively manage the battery pack, making informed decisions regarding charging, discharging, cell balancing, and safety protocols. This wireless approach introduces flexibility and convenience in battery management, potentially offering benefits in terms of ease of installation and maintenance in a variety of applications.
Advantages and Challenges of Wireless BMS
There are many possible advantages of wBMS. First and foremost, the lack of physical wire makes the design of the battery management system simpler and reduces the battery pack's overall weight, which is an important factor in electric vehicles where every gram counts. Additionally, the modular design of WBMS can increase the system's adaptability and scalability by making it simple to add or replace battery cells.
Additionally, wBMS might provide advantages in terms of dependability and safety. The risk of failures or short circuits is reduced by eliminating physical connections that can eventually corrode or break. Furthermore, wireless connectivity may enable more frequent and thorough data collection from individual cells, improving optimization and preventive maintenance.
Adoption of wBMS is not without difficulties. Making wireless communication reliable, especially in situations where interference may occur is a serious challenge that may be encountered. Additionally, since battery systems are essential parts of automobiles and energy systems, protecting this connection from potential cyber threats is of the utmost importance.
Overall, wireless BMS has promise for the future of battery management, but to realize that promise, its implementation must carefully address these issues. Current research and development in this field will put a lot of emphasis on the trade-offs between advantages and difficulties.
Solid-State Batteries
Introduction to Solid-State Battery Technology
Solid-state batteries (SSBs) are an emerging and highly promising innovation in the relentless pursuit of advanced energy storage solutions. In contrast to traditional batteries, SSBs feature a radical departure in design, as they make use of solid electrolytes as opposed to the more common liquid or gel electrolytes found in conventional battery technologies like lithium-ion cells. This groundbreaking shift in the battery paradigm holds tremendous potential for significant advancements in multiple key aspects, encompassing energy density, safety, lifespan, and the operational temperature range.
In terms of energy density, solid electrolytes facilitate the utilization of lithium metal anodes, which boast a notably higher theoretical capacity when compared to the graphite anodes that are prevalent in lithium-ion batteries. Moreover, the utilization of solid electrolytes offers an inherent advantage in terms of safety, as they are non-flammable, effectively mitigating the substantial risk associated with thermal runaway, which is a primary concern in conventional battery technologies.
Furthermore, the benefits of solid-state batteries extend to the operational temperature range. The use of solid-state electrolytes enables a potentially wider spectrum of temperatures within which these batteries can function efficiently. This expanded operational temperature range opens up new possibilities for deploying these batteries in diverse environments and applications, making them an attractive option with a profound impact on the energy storage landscape.
BMS Considerations for Solid-State Batteries
Solid-state batteries, despite their promising potential, bring forth novel challenges and factors that necessitate a reevaluation of Battery Management System (BMS) design and operation. While the core principles of battery management, such as State of Charge (SoC) estimation, cell balancing, and safety protocols, remain pertinent, the methods for achieving these functions may undergo substantial variations.
For example, because solid-state batteries exhibit distinct charge and discharge behaviors in comparison to liquid-electrolyte batteries, it might be imperative to formulate new models for precise SoC estimation. Similarly, cell balancing strategies might require adjustments due to the distinct self-discharge rates and varying internal resistance characteristics inherent to solid-state cells. The introduction of solid-state batteries into the energy storage landscape prompts an evolution in BMS design and operation, calling for innovative solutions tailored to the unique characteristics of this groundbreaking technology.
The thermal management demands associated with solid-state batteries could indeed deviate significantly from traditional batteries. Although these batteries may boast a broader operational temperature range and a reduced risk of thermal runaway, they might also necessitate a distinct approach to managing their thermal behavior, particularly due to potentially higher operating temperatures.
Furthermore, solid-state batteries may exhibit different failure modes compared to their liquid-based counterparts. This divergence in failure modes calls for the development of new techniques for fault detection and diagnostics. Consequently, Battery Management Systems (BMSs) tailored for solid-state batteries must be equipped to monitor and react to these distinctive operational characteristics and failure scenarios, necessitating an evolution in their design and functionality.
Next-Generation Energy Storage Solutions
Beyond Lithium-Ion: New Materials and Technologies
Lithium-ion batteries have unquestionably become the dominant technology in the realm of energy storage. However, the relentless quest for enhanced performance, greater safety, reduced costs, and environmentally friendly solutions is steering the exploration towards alternative materials and technologies, transcending the confines of lithium-ion.
A particularly promising avenue of research delves into multivalent-ion batteries, like magnesium-ion or aluminum-ion batteries. These innovative technologies hold the potential for achieving higher energy densities, primarily attributed to their capability to transport multiple electrons per ion. In contrast, sodium-ion batteries capitalize on the abundance and cost-effectiveness of sodium, offering an economically viable and sustainable solution for energy storage. These avenues signify the expanding horizons of energy storage research as we continue to seek more efficient, cost-effective, and environmentally conscious alternatives to conventional lithium-ion batteries.
Another noteworthy area of exploration in the field of energy storage centers around the advancement of metal-air batteries, with lithium-air and zinc-air batteries standing out as notable examples. These battery technologies hold the theoretical potential for significantly higher energy densities by leveraging oxygen from the atmosphere as the active material in the cathode. This innovative approach contributes to the reduction in both the weight and volume of the battery, promising substantial gains in energy storage efficiency.
Additionally, redox flow batteries are emerging as a subject of growing interest, particularly in stationary storage applications. Their appeal lies in their capacity to decouple energy and power capacity, potentially offering an extended cycle life and the unique ability to remain at a zero state of charge without experiencing degradation. This feature makes redox flow batteries an attractive choice for various energy storage requirements, further diversifying the landscape of energy storage solutions.
Energy Storage Integration with Renewable Energy
In the ongoing journey towards sustainable and resilient energy systems, the seamless integration of energy storage with renewable energy sources assumes a paramount role. Within this overarching framework, Battery Management Systems (BMSs) emerge as indispensable architects, orchestrating and navigating the intricate, dynamic interplay that characterizes the relationships between renewable energy sources, such as wind and solar, energy storage units, and the expansive electrical grid network.
Through their deft control over the charging and discharging processes of energy storage systems in harmonious alignment with the prevailing availability of renewable energy, BMSs wield a transformative influence. They are adept at capitalizing on this synergy to unlock the full potential of renewable energy utilization. Consequently, BMSs undertake the task of harmonizing and stabilizing the often variable output of renewable energy systems, thereby ushering in an era of enhanced reliability in renewable energy generation. Moreover, they contribute substantially to reducing dependency on conventional power grids or diesel generators, particularly during those challenging intervals characterized by scant renewable energy production, thereby enhancing energy resilience and sustainability.
In addition, a meticulously designed and executed Battery Management System (BMS) assumes the role of a proficient guardian, adept at mitigating the disruptive impacts of grid disturbances and preserving the unwavering reliability of the power supply. Its capabilities extend to a prompt and responsive reaction to fluctuations in power generation and the ever-evolving demands of the grid. This is instrumental in rendering support to the grid through activities such as frequency regulation and the provision of various other crucial grid support services, underlining the pivotal position of BMS in energy management and grid stability.
To elevate these intricate interactions to an optimal level, advanced BMS systems have the potential to harness the capabilities of Machine Learning (ML) algorithms. These algorithms are geared towards the predictive realm, foreseeing aspects such as renewable energy production through a meticulous analysis of weather forecasts, grid demand by tapping into historical data patterns, and the implementation of judicious storage control strategies. These control strategies, framed around the state of health and the state-of-charge of the battery system, hold the promise of ushering in a new era of unprecedented energy management, where precision and efficiency reign supreme.
IoT and Smart Grid Integration
BMS in Smart Grids
The Battery Management System (BMS) is becoming more and more important in determining how energy is produced, stored, and consumed as the era of smart grids approaches. The production and distribution of electricity are improved in terms of efficiency, dependability, economics, and sustainability through the smart grid, a modernized electrical system that makes use of information and communications technologies.
The BMS can function in this situation as an intelligent agent that interacts with the smart grid to transmit information about the battery's SOC, SoH, charging/discharging rates, and other important characteristics in real time. Peak shaving, load leveling, and demand response tactics are supported, allowing for more effective and adaptable energy management.
To illustrate, consider high-demand scenarios, commonly observed during peak hours, where the intricacies of a smart grid system could seamlessly communicate with the Battery Management System (BMS). This intelligent dialogue might entail the smart grid signaling the BMS to initiate the discharge of stored energy into the grid. Such an action effectively alleviates the demand for power generation from the more costly and less energy-efficient peaking power plants. In essence, this dynamic interaction serves as a strategic solution that contributes to grid stability and cost optimization.
Vehicle-to-Grid (V2G) Technologies
Furthermore, the realm of Vehicle-to-Grid (V2G) technology elevates this synergy between the BMS and the grid to a higher plane. This innovative approach transcends the traditional role of electric vehicles (EVs) as mere energy consumers and envisions them as potential energy storage reservoirs, primed to discharge energy back into the grid whenever the need arises.
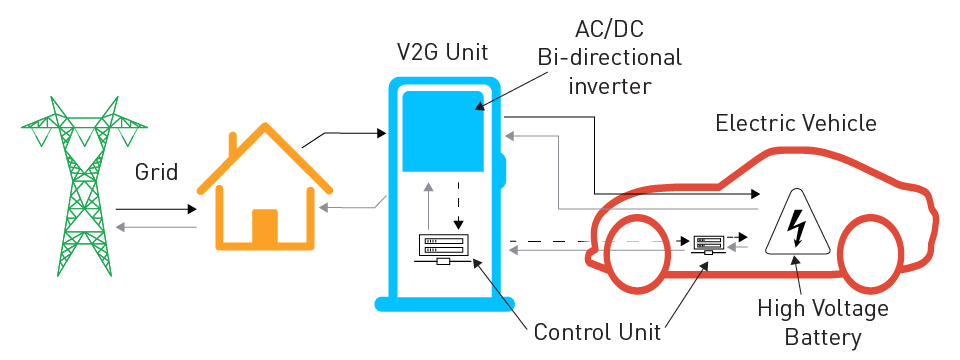
Figure 3: V2G
This dual-directional energy transfer is expertly facilitated by the Battery Management System (BMS), which exercises precise control over the intricate dance of charging and discharging. The BMS operates with a primary goal in mind: safeguarding the battery's well-being by meticulously averting the perils of overcharging and deep discharging. It accomplishes this while ensuring the seamless and efficient transfer of energy. In the context of Vehicle-to-Grid (V2G) scenarios, the BMS takes on an added layer of complexity. It's entrusted with the task of harmonizing the mobility requirements of the vehicle, ensuring that adequate charge levels are maintained to cater to the driver's needs and expectations. This dual role underscores the versatility and adaptability of the BMS in the dynamic realm of V2G technology.
A potential answer to the problem of intermittent renewable energy sources is the use of V2G technologies in smart grids. For instance, EVs could charge when there is a high production of renewable energy (such as on sunny or windy days) and discharge their stored energy when there is low production of renewable energy, thus acting as mobile energy storage units that support grid stability and the integration of renewable energy.
Additionally, V2G can offer auxiliary services to the grid, such as frequency control and voltage control, enhancing the power system's resilience and dependability. The potential of V2G technology is enormous, offering a future in which vehicles play a crucial role in the larger energy ecosystem, made possible by intelligent and flexible BMS, as the EV industry continues to grow.
Safety and Security Considerations for Future BMS Technologies
With the ongoing evolution of Battery Management Systems (BMS), featuring increasingly intricate functionalities and capabilities such as Artificial Intelligence (AI), Machine Learning (ML), and Internet of Things (IoT) connectivity, there arises a pressing need to delve into the safety and security dimensions inherent to these technological advances.
Safety in the context of BMS technology takes center stage, primarily focused on a proactive stance aimed at averting potential battery failures that could escalate into hazardous scenarios, notably thermal runaway. The forthcoming generation of BMSs is poised to usher in a new era, equipped with state-of-the-art sensing technologies and predictive algorithms that empower them to proactively anticipate potential safety risks and undertake preventative measures. For instance, AI and ML technologies are poised to play a pivotal role in identifying subtle deviations in battery operation that may serve as precursors to an impending failure. Moreover, the advent of wireless BMS opens the door to remote monitoring and diagnostics, enabling the rapid detection and resolution of safety concerns, and effectively setting the stage for a new era of safety and reliability within the domain of battery management.
But these features also bring about fresh cybersecurity difficulties. There are more potential avenues of entry for cyberattacks due to increased connectivity and the usage of sophisticated algorithms. The safety and functionality of the entire system that the battery powers may be impacted by a corrupted BMS that provides inaccurate information about the battery's condition. For instance, if the SOC reading is altered, it may lead to deep draining or overcharging, both of which can harm the battery and pose safety risks.
Future BMS solutions will need to include strong cybersecurity protections to solve these challenges. Anomaly detection methods, secure communication protocols, encryption, and routine software upgrades to fix recently found flaws are a few examples. These security measures must be made to withstand both internal and exterior risks, such as unintentional configuration errors or software defects, as well as external threats like hacker attempts.
Furthermore, it is imperative to guarantee that these security measures do not impede the fundamental safety functionalities of the BMS. The BMS must retain the capacity to respond swiftly to critical safety occurrences, even when confronted with a cyber assault or system malfunction. Achieving this necessitates a meticulous equilibrium between safety, security, and performance, calling for a multidisciplinary strategy that unites proficiency in battery technology, computer science, and systems engineering.
In summary, as BMS technologies progress in sophistication and interconnectedness, the pivotal factors of safety and security will assume an ever more conspicuous role in their conception and function. This multifaceted challenge necessitates continual research and the collaborative synergy of scientists, engineers, policymakers, and industry participants. Through the combined endeavors of these stakeholders, we can ascertain that forthcoming BMS technologies not only furnish enhanced performance and capabilities but also uphold the elevated safety and security benchmarks anticipated by users and society as a whole.
直接登录
创建新帐号